Executive summary
What happened
On the morning of 2 October 2022, a Robinson Helicopter Company R22 Beta II, registered VH‑RAS, departed Koorda, Western Australia for a private flight, with the pilot and one passenger on board. About 6 minutes later, the helicopter impacted terrain, inverted, about 13 km to the southwest of the departure point. The helicopter was destroyed, and both occupants were fatally injured.
What the ATSB found
Recorded flight data showed that, during cruise, the helicopter’s altitude increased by about 100 ft and then rapidly descended, almost vertically, before colliding with terrain inverted. The ATSB found that the helicopter sustained an in-flight break-up at about the time it rapidly descended. The site and wreckage examination identified signatures indicative of a low-g and/or low rotor RPM/rotor stall condition, however, the circumstances preceding this could not be determined.
Dual flight controls were fitted in a position that was occupied by the passenger. When carrying passengers, the helicopter manufacturer recommends removing the passenger‑side controls to avoid inadvertent bumping or interference.
The ATSB also found that the pilot had not disclosed their use of a prescription medication or the associated medical condition to the Civil Aviation Safety Authority. This precluded a specialist assessment of the aeromedical significance of the medication’s use and the underlying conditions for which it was prescribed.
Safety message
Low-g and low rotor RPM/rotor stall conditions can be catastrophic for helicopters with semi‑rigid rotor heads. A pilot’s ability to identify the condition and promptly apply the correct flight control inputs is vital to effective recovery and continued safe operation. It is also particularly important that the dual flight controls be removed before flight to avoid inadvertent passenger interference. Where the dual controls cannot be removed, the passenger should be fully briefed to keep their hands and feet clear.
This accident also highlights the importance of pilots reporting relevant medical conditions and the use of medications to their designated aviation medical examiner. A full understanding by the Civil Aviation Safety Authority’s aviation medical specialists of a pilot’s medical conditions, and use of medications, enables management of the risk for both the individual and flight safety overall.
Further, recording devices have long been recognised as an invaluable tool for investigators in identifying the factors behind an accident, and their contribution to aviation safety is irrefutable. While not required by regulations, owners and operators should consider the benefits of installing such devices.
The investigation
Decisions regarding the scope of an investigation are based on many factors, including the level of safety benefit likely to be obtained from an investigation and the associated resources required. For this occurrence, a limited-scope investigation was conducted in order to produce a short investigation report, and allow for greater industry awareness of findings that affect safety and potential learning opportunities. |
The occurrence
Flight from Jandakot to Koorda
On 1 October 2022, a Robinson Helicopter Company R22 Beta II, registered VH‑RAS, departed Jandakot Airport, Western Australia for a private flight to an airstrip at Koorda (Figure 1 inset). The helicopter and several aeroplanes were being used to participate in a group social flying weekend, with the pilots and their passengers following a common itinerary but operating independently. The helicopter was being operated under the visual flight rules.[1]
The group arrived around midday at Koorda, and a witness recalled assisting the pilot of VH-RAS to fuel the helicopter with avgas[2] from 2 full 10 L plastic fuel containers, which they had been given by the pilot to drive to Koorda from Jandakot. This fuel was loaded into the main fuel tank. This witness also observed the pilot conduct a fuel drain during the pre‑flight inspection the next morning.
Return flight to Jandakot
On the morning of 2 October 2022, the group departed at staggered times from Koorda, on the return flight to Jandakot. VH-RAS departed at about 1140 local time, with the pilot and one passenger on board. It was reported that the pilot intended to refuel at Northam aerodrome, about 117 km to the south-west of Koorda.
When VH-RAS did not arrive at Jandakot as expected, and after confirming it had not landed at Northam, the helicopter was reported as overdue at about 1500 and a search was commenced. The search was coordinated by the Australian Maritime Safety Authority’s Joint Rescue Coordination Centre. The wreckage was subsequently located at about 1600 on a dry salt flat in the Cowcowing Lakes region, about 13 km south-west of Koorda (Figure 1). The helicopter was destroyed, and both occupants were fatally injured.
Figure 1: Flight from Jandakot to Koorda and from Koorda on the morning of the accident
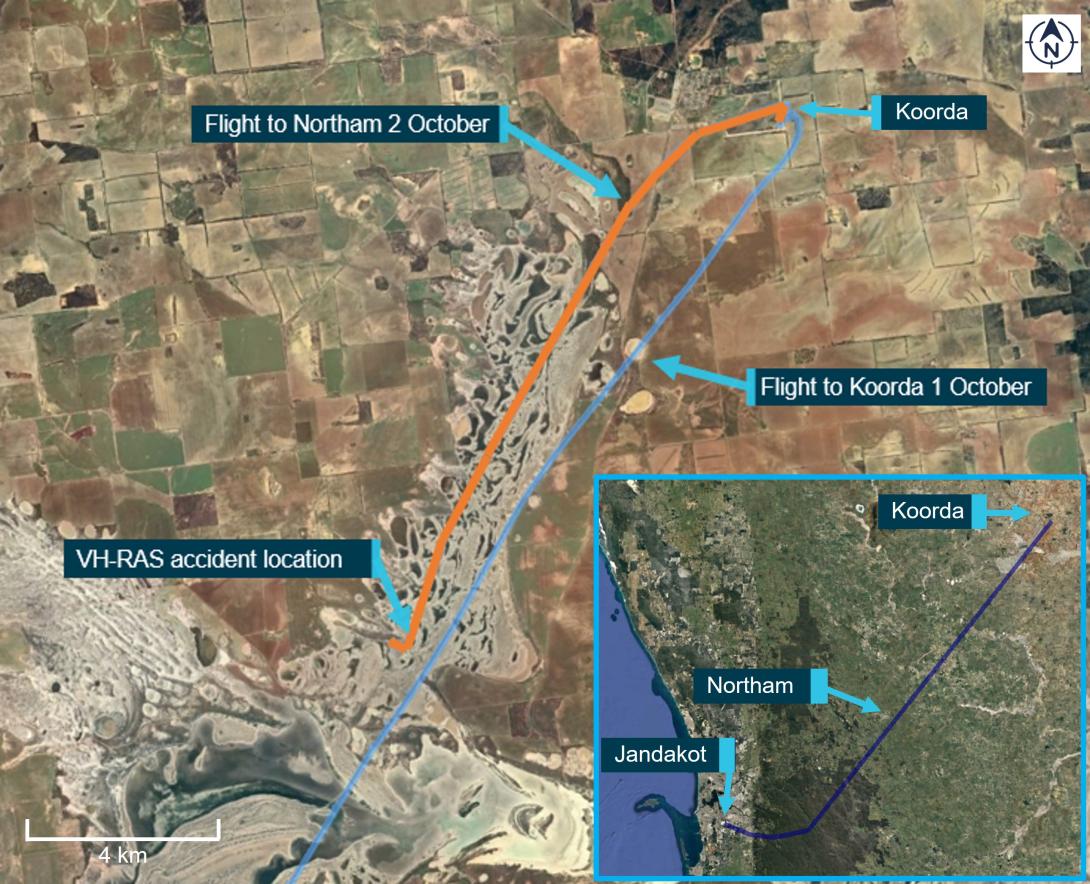
Source: Google Earth and handheld GPS data, annotated by the ATSB
Context
Pilot information
General
The pilot was the owner of VH-RAS and held a private pilot licence for both aeroplanes (since 1976) and helicopters (since 2006). Their last flight review, conducted in VH‑RAS, was completed 11 January 2021.
The pilot held a Class 2 Aviation Medical Certificate valid to 22 January 2024, which included restrictions requiring the wearing of distance vision correction and that reading correction must also be available while exercising the privileges of the licence. The pilot reportedly did not wear glasses while flying. However, their flight instructor and other pilots who flew with them, advised that they had not noticed any impediment with the pilot’s ability to operate the helicopter without wearing distance vision correction or at other times when reading the flight instruments.
Aeronautical experience
At their last medical in January 2022, the pilot reported that they had accrued about 3,000 hours total aeronautical experience (combined aeroplane and helicopter). It was also reported that the pilot no longer maintained their logbook, which prevented an accurate determination of their experience specific to aeroplanes and helicopters.
There were 16 entries on the current maintenance release, indicating the pilot typically flew the helicopter about twice per month, for about 20 minutes each time.[3] A review of previous maintenance releases determined that the pilot had operated the helicopter for a total of 77 hours since its purchase in 2016.[4]
The pilot was reported to have regularly flown the helicopter accompanied by an experienced aeroplane commercial pilot and instructor, who also held a helicopter licence. Those flights would typically occur each month and involve about 45-minutes[5] of skill‑based flying practice, consisting of general handling, hovering, hovering turns and slope landing practice. No simulated emergencies or abnormal operations were practiced during those flights. In addition to those flights, they would do short flights to properties in the Perth hills or to nearby aerodromes to participate in social ‘fly-in’ breakfast events.
The pilot’s January 2021 helicopter flight review was performed by an experienced helicopter instructor and flight examiner who had conducted a significant amount of the pilot’s training and testing over the years. The flight review included simulated engine failures, autorotation[6] to confined areas, main rotor under speed recoveries and stuck tail rotor pedal emergencies.
In addition to the flight review, the instructor recalled accompanying the pilot on other occasions during the period since January 2021. During this, the pilot had the opportunity to practice simulated emergencies under the instructor’s supervision.
The aviation community at Jandakot held the accident pilot in high regard and recognised them as somebody who had natural aptitude as both an aeroplane and helicopter pilot.
Recent history
The pilot was reported to typically retire to bed about 2100 and wake about 0500 most days. They were described consistently as being very health conscious, having exercised regularly and maintained very good overall health.
Members of the flying group that had spent time with the pilot during the weekend recalled them being their normal self, including prior to departing Koorda the morning of the accident. The pilot was reported to have slept normally, was well rested and had eaten breakfast with the group prior to arriving at the airstrip. There was no evidence to indicate the pilot was unwell nor experiencing a level of fatigue known to affect performance.
Passenger information
The passenger held a Student Pilot Licence (Aeroplane), with about 15 hours of flight experience in a Cessna Aircraft Company 152. They also held a valid Class 2 Aviation Medical Certificate, which included restrictions requiring the wearing of distance vision correction. It was stated that this was the passenger’s first flight in a helicopter. The passenger was reported to be well and that they appeared normal during the weekend, including prior to departing Koorda.
Helicopter information
General
VH-RAS (Figure 2) was a 2-seat Robinson Helicopter Company (RHC) R22 Beta II helicopter, serial number 4617, powered by a Textron Lycoming O-360-J2A, 4-cylinder carburetted piston engine. It was manufactured in the United States in 2013 and first registered in Australia the same year. It was purchased by the pilot in 2016 and had been maintained by the same maintenance organisation since that time. The helicopter was to undergo a periodic inspection every 100 hours or 12 months, whichever came first. The last periodic inspection had been completed in November 2021, with the current maintenance release issued at that time. At the time of the accident, the helicopter had accrued about 13 hours since the periodic inspection and 2,080 hours total time-in-service.
The helicopter was equipped with an intercom system and headsets, allowing those on board to talk to each other during flight. This would allow for clear communication between the pilot and passenger in the event of an abnormal situation.
Figure 2: VH-RAS
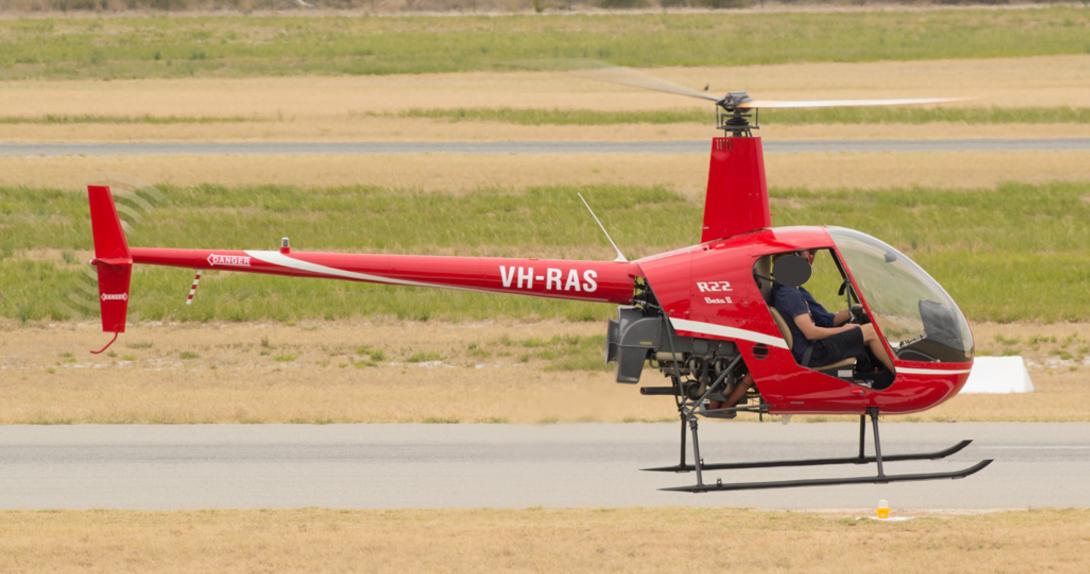
Source: Dallas Presser, modified by the ATSB
Rotor head design
The 2-blade main rotor assembly is a semi-rigid rotor head, otherwise known as a teetering rotor head (Figure 3). Bolts secure the rotor blades to the main rotor hub at the coning hinges.[7] The teetering head allows the rotor disk to take up an attitude appropriate to the flight condition, with the coning hinges allowing the blades to achieve the optimum coning angle appropriate for the flight and loading conditions. This design permits a lighter blade structure than would otherwise be required.
During stopping and starting of the main rotor, when the revolutions per minute (RPM) are low, the spindle tusks rest against the droop stops, which are mounted near the top of the main rotor mast. The droop stops restrict the drooping of each blade to prevent contact with the tailcone at low rotor RPM. As the main rotor RPM increases, the blades become rigid and straighten due to rotational forces, and the tusks shift off the droop stop as the blades lift. During normal flight, the rotor is free to teeter and flap around its designed flight axis via the teeter hinge, while polyurethane teeter stops limit the degree of teetering to protect the mast from direct contact with the main rotor blades. The main rotor system is also fitted with pitch change links, which connect the swashplate to the pitch horn and transmit the flight control inputs to the main rotor blades.
Figure 3: R22 main rotor head assembly
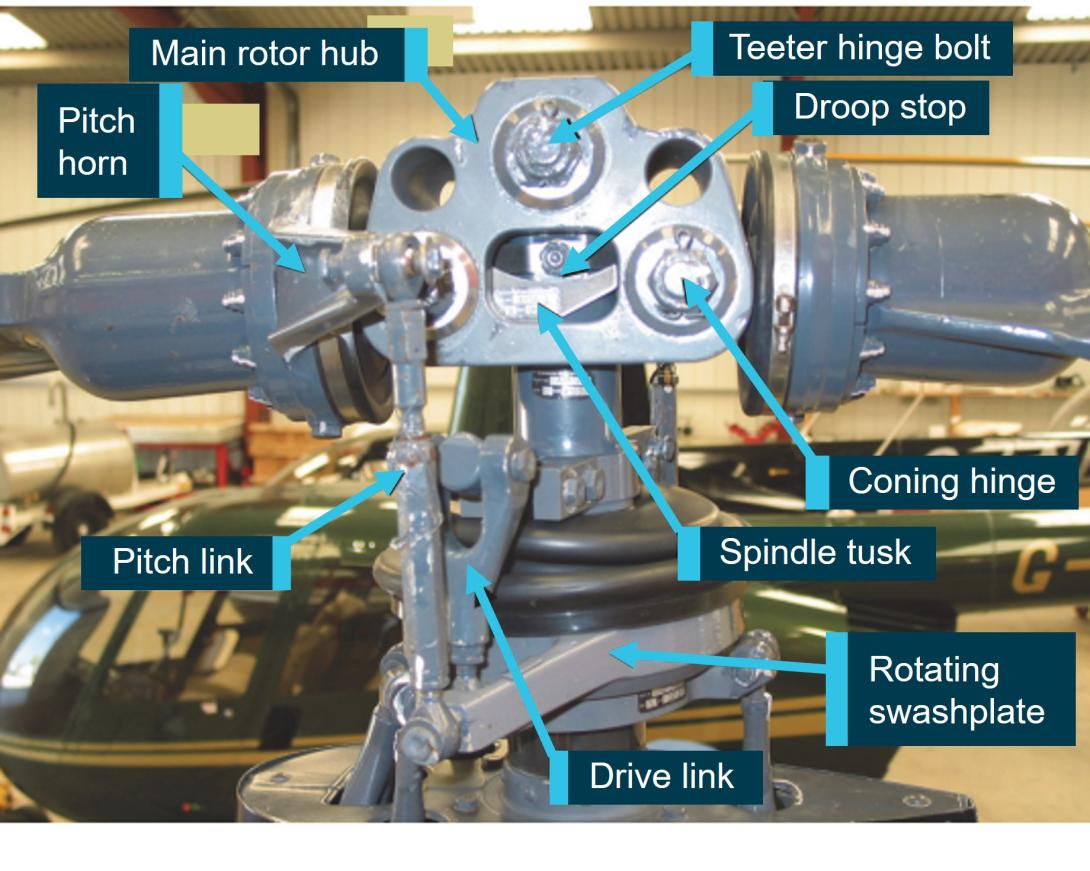
Source: United Kingdom Air Accidents Investigation Branch, annotated by the ATSB
Flight controls
The tail rotor pedals change the pitch of the tail rotor blades, and therefore the thrust of the tail rotor system, which provides directional control. The collective lever controls the amount of thrust (lift) produced by the main rotor disc. Raising or lowering the collective lever will raise or lower the swashplate,[8] which will alter the pitch on both main rotor blades to increase or decrease the main rotor thrust. The collective lever also incorporates a twist grip to provide the pilot with full manual control of the engine throttle. The cyclic[9] control tilts the main rotor disc to point the rotor thrust in the desired direction of flight. Fore‑aft movement of the cyclic provides the longitudinal (pitch) control of the main rotor disc. Left‑right movement of the cyclic provides lateral (roll) control of the main rotor disc.
The helicopter was fitted with quick-disconnect dual flight controls (exemplar shown in Figure 4).[10] This consisted of duplicated collective and tail rotor pedal controls for both the pilot (right) and passenger (left) seating positions, and an additional control arm to the left of the T-bar cyclic control, enabling the cyclic control of the helicopter to be transferred between positions. In that configuration, the T-bar cyclic control would tilt down to be directly in front of the person controlling the helicopter. The opposite side cyclic control would still be connected and in front of the other seating position, but in a significantly higher position than normal (Figure 4 inset). The dual controls for the left seat position could be easily removed for passenger carrying operations. Notably, the R22 Pilot’s Operating Handbook (POH) included the caution:
…remove left seat controls if person in that seat is not a rated helicopter pilot.
Figure 4: Dual flight controls, with insert showing deflection when being operated from the right
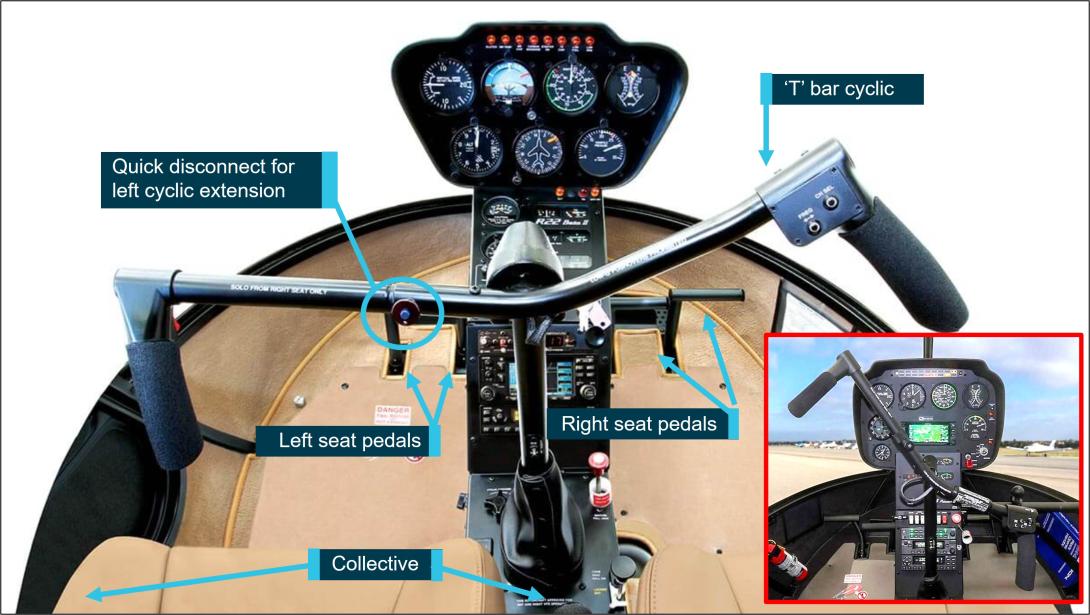
Note: While the insert image is from a R44 helicopter, it demonstrates the position of the opposite cyclic grip when the T-Bar is oriented to the pilot sitting in the right seat, with similar see-saw orientation when being held by the left seat pilot.
Source: Robinson Helicopter Company, annotated by the ATSB
Fuel system
The fuel system consists of a main tank (left side, 69 L) and an auxiliary tank (37 L, right side). Fuel is gravity-fed (no pumps) via the gascolator to the carburettor.
Engine governor system
Under normal conditions, the governor senses engine RPM and makes adjustments to the throttle control to maintain a constant engine RPM, which leads to a constant rotor RPM in flight. It can be selected on or off using the toggle switch on the right seat collective. The POH stated that the governor may not prevent over- or under-speed conditions generated by aggressive flight manoeuvres. In the event of malfunction, the pilot can override the governor and manipulate the throttle to maintain engine RPM, until the governor can be selected off, or rendered inoperative by pulling the circuit breaker.
Carburettor heat system
The helicopter was fitted with a carburettor heat system, which directed hot air collected from a scoop installed on the engine exhaust system, via a duct, to the engine induction air box. Within the airbox was a sliding guillotine-type valve to proportion the mix of cool and heated air. The pilot could monitor the temperature of the carburettor air using the carburettor air temperature gauge on the instrument panel console.[11] The carburettor heat control knob was situated aft and rear of the cyclic, with ‘down’ being no heat and ‘up’ providing full heat, or anywhere in between as selected by the pilot. This heated air prevented the temperature within the carburettor from dropping to at or below the freezing point of water. While the increase in carburettor air temperature would result in a small reduction in power, this could be countered by increasing throttle and manifold pressure.
The helicopter also had a carburettor heat assist system, which automatically applied carburettor heat when lowering the collective, generally for descent, to reduce pilot workload. The pilot could override the heat assist. In addition, a latch was provided at the carburettor heat control knob to lock the heat assist off when not required.
Weight and balance
Weight and balance for the accident flight was calculated using the approved weight and balance record, together with the estimated fuel load, occupant weights and recovered luggage. To calculate the fuel on board departing Koorda, the ATSB used scenarios where the helicopter was fully‑fuelled prior to departing Jandakot and the average fuel consumption (as specified by RHC) was between 7 and 10 US gallons per hour (26–38 L/hr). Using those rates, the ATSB estimated that, on engine start at Koorda, the helicopter had between 40‑54 kg (55–75 L) of fuel onboard, which included the 20 L added the day before.
Irrespective of the fuel load departing Koorda, the helicopter’s lateral and longitudinal centre of gravity remained within the limits published by the manufacturer. However, the helicopter would have been at about its maximum gross operating weight if departing Koorda with 40 kg of fuel but could have exceeded the maximum gross weight by about 15 kg if departing with 54 kg of fuel. Despite this, as the helicopter was observed to have departed normally, and reached a cruise altitude, this would suggest that the overall weight did not significantly reduce the helicopter’s performance.
Meteorological information
Bureau of Meteorology forecast and analysis
The graphical area forecast prepared by the Bureau of Meteorology indicated visual meteorological conditions[12] were expected during the flight to Jandakot. Winds were forecast to be generally east-south-easterly below 5,000 ft above mean sea level, between 15–20 kt. There were no SIGMET[13] or AIRMET[14] warnings applicable to the flight.
An analysis prepared by the Bureau of Meteorology indicated a high-pressure system was situated to the south‑west of Western Australia, producing moderate east to south‑easterly winds in the vicinity of the accident site. Satellite imagery and measurements from the weather station at Cunderdin (about 80 km south of the accident site) indicated there was scattered cloud[15] in the vicinity of the site, with bases approximately 4,500–5,000 ft. There was the possibility of some thermal turbulence as the surface temperature and cloud base increased during the day, with a well-mixed air layer below the cloud. However, the analysis did not identify the existence of any hazardous weather phenomena in the vicinity of the accident site.
The air temperature at 2,500–3,000 ft was estimated to be about 10–12 °C, with a dewpoint[16] of about 6–8 °C. According to the Civil Aviation Safety Authority Carburettor icing probability chart, this temperature and dew point were within the ‘serious icing – any power’ envelope.
Pilot reports
Pilots of the other aircraft in the group reported good flying conditions during the flight back to Jandakot. Those reports were generally consistent with the Bureau of Meteorology’s analysis. There was slight variation in the pilots’ estimates of the cloud bases during their flights, ranging between 3,000–4,500 ft. Several pilots who departed Koorda that morning reported encountering some turbulence over the Cowcowing Lakes area. Most described the turbulence as mild, but one pilot reported an instance of ‘moderate’ turbulence,[17] an updraft of sufficient magnitude to startle their passenger and dislodge loose items in the cockpit.
Recorded information
The helicopter was not fitted with a cockpit voice recorder, flight data recorder or cockpit camera, nor was it required to be. Neither the pilot nor the passenger’s mobile telephones were identified at the accident site.
Sources of data
A handheld GPS receiver and lanyard was recovered at the accident site and data was successfully downloaded. A review of the data found that during the accident flight, data was recorded at intervals varying between 1 and 19 seconds, with shorter intervals representing periods where the track and speed calculated by the GPS was rapidly changing. The information logged included GPS-calculated position, track, groundspeed, and altitude.
While no mobile telephones were found, the passenger was using the OzRunways[18] application installed on their iPhone during the flight. The application was using the mobile telephone network to transmit data to the OzRunways’ servers every 5 seconds, which included the current position, track, groundspeed and truncated altitude in increments of 100 ft.[19]
Correlation of data
There was a strong correlation between the track log from the handheld GPS and the data transmitted by the iPhone to the OzRunways’ servers. The only significant discrepancies were during the initial climb with the handheld GPS-calculated altitude lagging behind the altitude data transmitted from the iPhone and during the last 10 seconds of recorded data (altitude, groundspeed, and position). Analysis of the last 10 seconds of data indicated several of the positions calculated by the handheld GPS required groundspeeds that exceeded the helicopter’s performance capabilities. During that period, any rapid changes in the satellite constellation used by the GPS could adversely affect the accuracy of the calculated position. The position calculated by the iPhone used additional sensors and GPS frequency bands, and those positions were more consistent with the helicopter following that trajectory during the descent.
Analysis of recorded data
The elevation of Koorda airstrip was about 1,060 ft above mean sea level. The handheld GPS started recording position information at 1136:32 local time. At 1139:50, the track log of the GPS indicated that the helicopter was airborne and on initial climb. Soon after, the OzRunways application on the iPhone started transmitting data, also consistent with the helicopter being airborne.
There was a close correlation between the 2 tracks and groundspeeds. The helicopter’s airspeed during the initial stages of climb was estimated to be between 60 and 65 kt,[20] which was consistent with the helicopter manufacturer’s recommended climb speed. The estimated airspeed increased progressively towards about 80 kt during the latter stages of the climb. About 3 minutes 40 seconds after take-off, the iPhone and GPS altitude stabilised at about 2,700 ft (refer Appendix A for graphed data).
During the latter stages of the climb and the initial cruise, the pilot’s tracking of the helicopter towards Northam closely matched the track required[21] and any track error was generally less than 5°. This was similar to the tracking performance achieved by the pilot during the outbound flight on the previous day.
About 1 minute after reaching 2,700 ft, the tracking accuracy towards Northam began to reduce (measured as the calculated difference between the track required and track made good).[22] About 20 seconds later (1144:48), the altitude indicated by the iPhone had increased by a 100 ft increment to 2,800 ft (and the handheld GPS altitude also increased by a commensurate amount), with an estimated airspeed of 83 kt.
About 45 seconds after reaching 2,800 ft and with an airspeed of about 76 kt (at 1145:33), the iPhone data indicated that the helicopter had departed abruptly from controlled flight, descending from 2,800 ft to 1,300 ft over a 10-second interval (a rate of descent of about 9,000 ft/min). The helicopter subsequently collided with terrain (at an elevation of 930 ft), about 6 minutes after becoming airborne at Koorda.
Data transmitted by the iPhone (blue) and data from the handheld GPS (green) is depicted in Figure 5 and Figure 6.
Figure 5: Correlated track data for VH-RAS from Koorda to the accident site
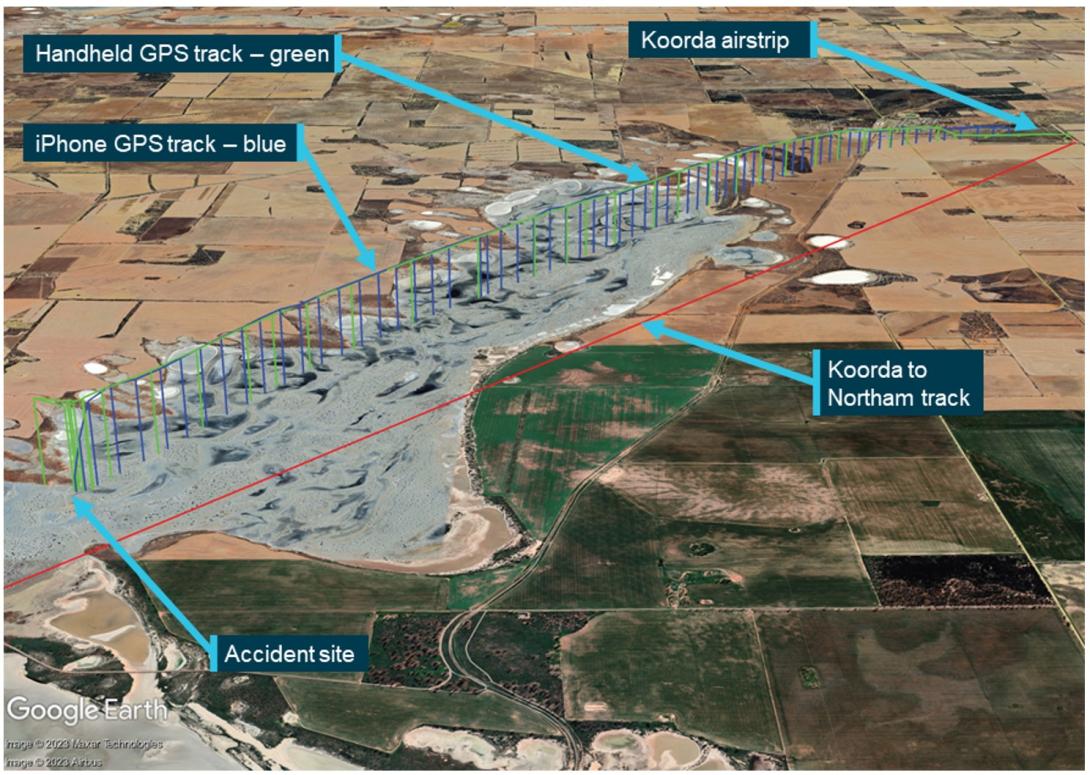
Source: Google Earth, annotated by the ATSB
Figure 6: iPhone track data, truncated altitude, and estimated airspeed for VH-RAS during last the 2 minutes of flight
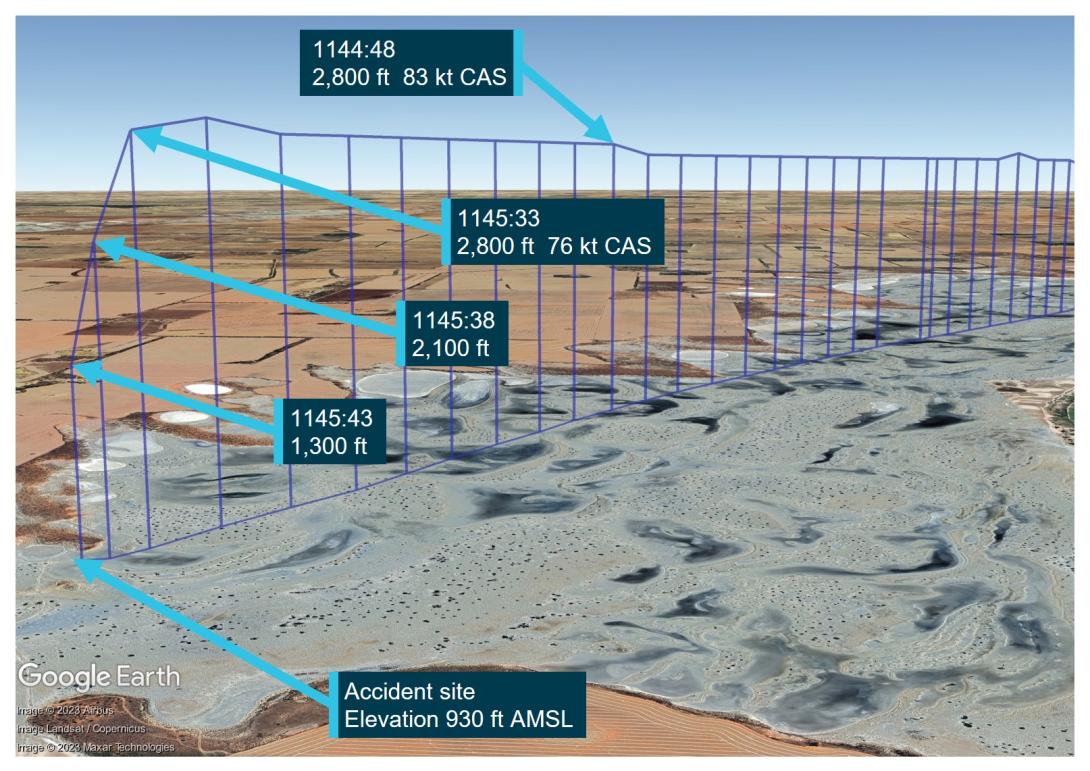
Source: Google Earth, annotated by the ATSB
Helicopter onboard camera
RHC introduced cockpit video cameras, which have been standard on new R66 and R44 helicopters since early 2021 and 2022 respectively and are an optional retrofit to in-service helicopters of both types. At the time of publication of this report, it remained optional for new R22 helicopters, with retrofit available to most in-service R22s. The forward-facing camera records video (encompassing a view through the windshield, pilot controls and the instrument panel), intercom audio, radio transmission and GPS data. RHC advised the recordings (up to 10 hours) can be used as a training tool, maintenance aid, or aerial-tour souvenir.
The recording could also assist with occurrence investigations by allowing investigators to understand the circumstance/s that precede an accident, particularly when there are no survivors or witnesses. In turn, this aids the identification of important safety issues.
Wreckage and impact information
Wreckage distribution
The helicopter collided with terrain inverted, on a dry salt flat, on an easterly heading. A small distance below the salt crust was loose muddy sand and the water table was close to the surface. The main rotor head, with blades attached, and the top portion of the mast had separated but were located alongside the fuselage (Figure 7). One main rotor blade had fractured, with the outboard section located about 3 m from the main rotor assembly. The tailcone, including the tail rotor assembly, was attached to the fuselage. The horizontal and vertical stabiliser assembly had separated and was located about 6 m from the tailcone. The auxiliary fuel tank bladder was intact, however, the main tank bladder had ruptured due to impact forces. There was no fire.
Distribution of the wreckage and ground scars were consistent with an almost vertical descent and little to no rotation of the main rotor blades. Both occupants were found secured in their respective restraints, however, due to the high descent rate and inverted orientation, the impact was not considered survivable.
Figure 7: Accident site and wreckage
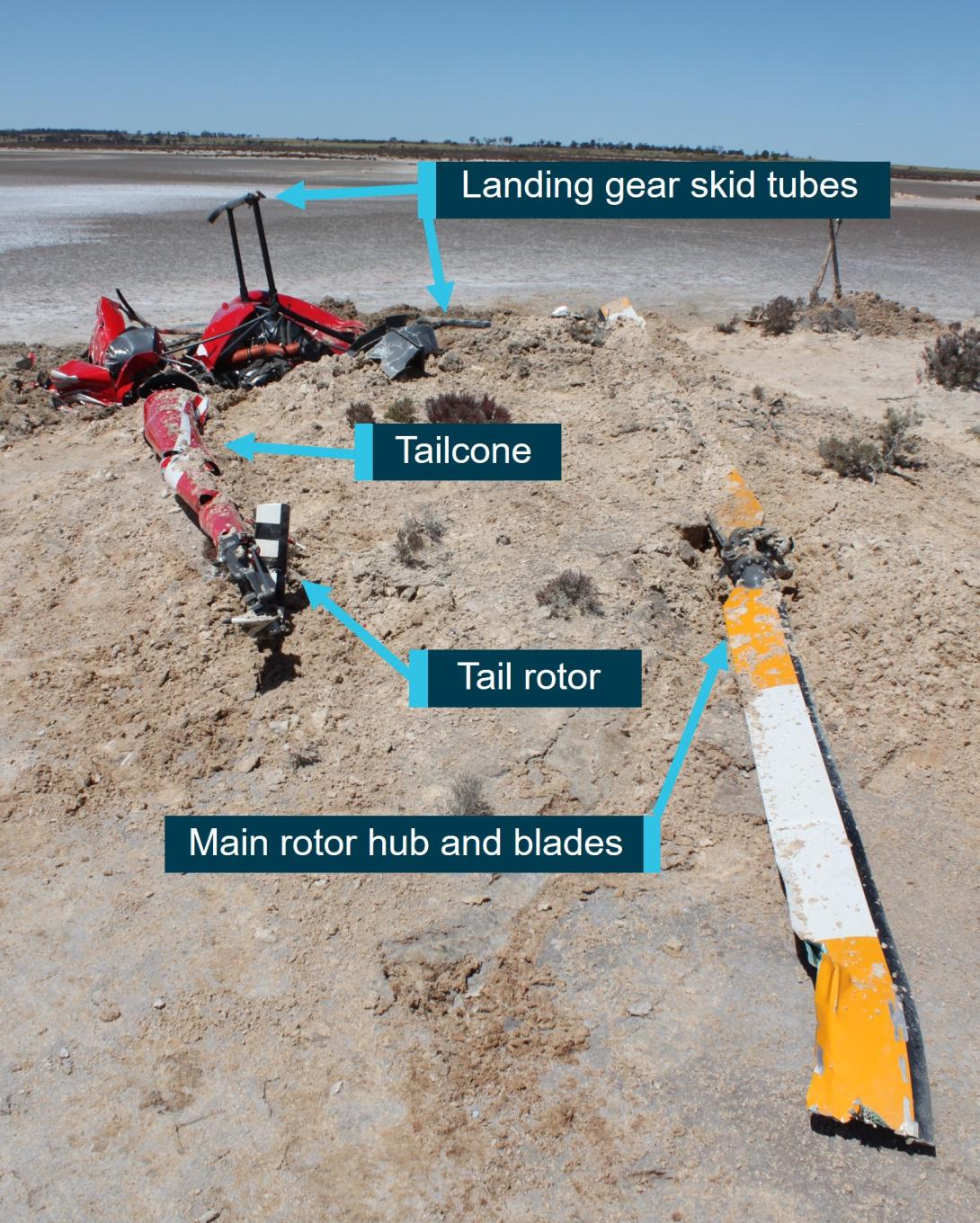
Source: ATSB
Wreckage examination
Detailed examination of the wreckage identified continuity of the flight and engine controls, with all fractures consistent with overstress failure, however, distortion to the fuselage precluded determining engine control position prior to impact. There was nil evidence of birdstrike found in the wreckage or the surrounding area. The examination further identified the following:
Powerplant
There was no evidence of a restriction or blockage to any part of the air induction system. Notably, the air intake SCEET hose[23] was in good condition with nil delamination and the air box filter was clear. The governor switch (located at the end of the right seat collective) was in the OFF selection. Although this may not necessarily reflect the position of the switch prior to the accident due to the damage sustained to the helicopter during the impact sequence. In addition, the governor circuit breaker was noted to be in the operational selection (not pulled due to failure or troubleshooting).
The carburettor heat selector was fully down (no heat) and the carburettor heat assist lockout latch was unlocked. However, it was possible these positions were altered during the impact sequence. Therefore, the actual positions prior to the impact could not be determined. The air box carburettor heat slider was about mid travel, but impact forces and fuselage distortion pulled the control cable, preventing determination of the pre-impact position. Some scoring to the alternator fan backing plate and oil cooler was indicative that the engine may have been rotating at impact, although the damage was not consistent with the engine operating at full power.
Drivetrain
There was no evidence of pre-existing defects to the drive shafts, drive belts or sheaves. The drive belt tensioning clutch actuator had fractured in overstress, however, the actuator setting was consistent with normal operation and stretch of in-use belts. The main rotor transmission case had fractured open on the forward right side, but there was no evidence of failure of the transmission internal gears. The ATSB could not determine if the fracture was sustained during extreme teetering (refer to section titled Extreme teeter and mast bumping) or the impact.
Main rotor system
Damage to the main rotor head components (refer Figure 8 for main rotor head components detail) included:
- both main rotor pitch change links had fractured, in overstress, at the upper rod end thread
- both spindle tusks had fractured and the tusks were not recovered
- the teeter stops had fractured in the centre due to severe impact forces from the spindles, the lower halves had been liberated and impact damage was visible on the mast.
The mast had fractured near the swashplate, with no evidence of pre-existing fatigue. The fracture location was coincident with impact from a pitch horn that was free to rotate down and impact the mast, following pitch link failure. The fractured main rotor blade exhibited red paint transfer and damage consistent with it striking the forward fuselage with low energy and wrapping under the cabin.
Figure 8: Main rotor head and mast damage
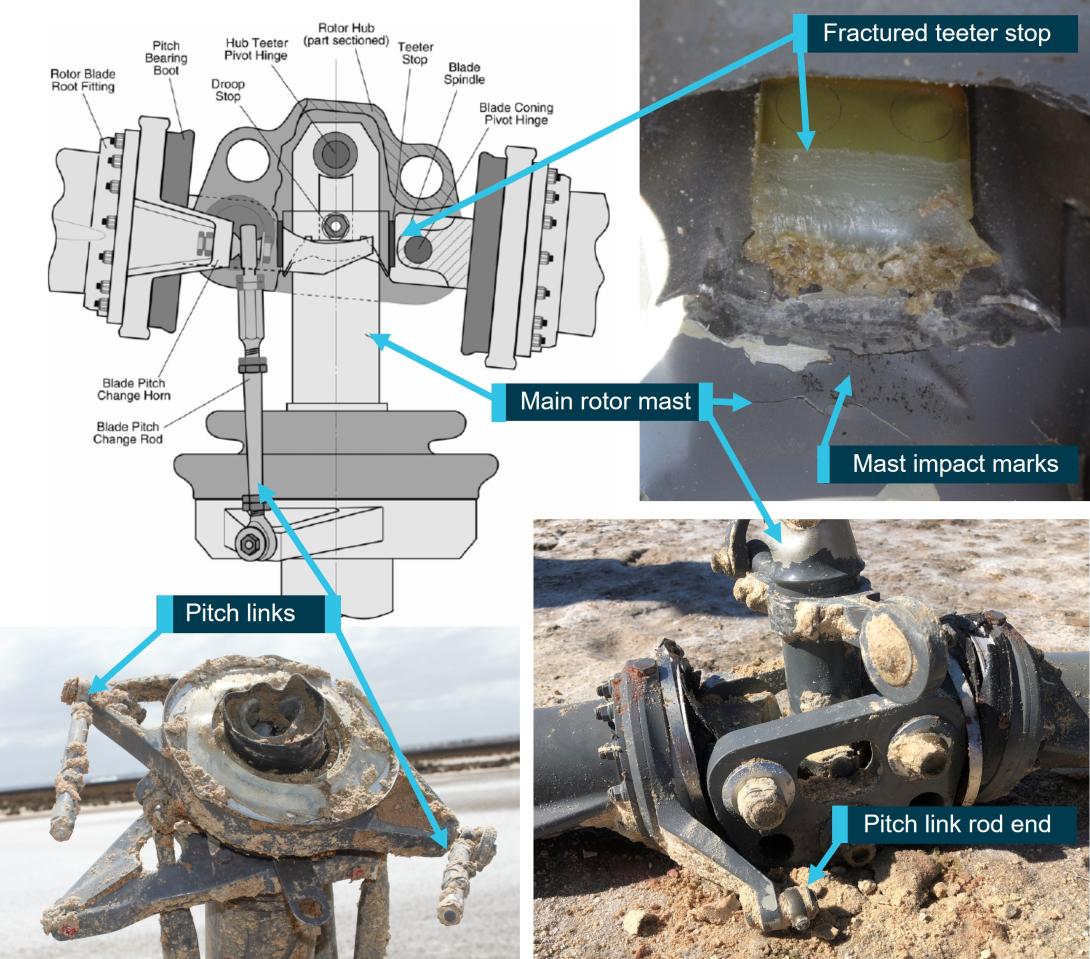
Note: Diagram of typical R22 main rotor head showing VH-RAS fractured pitch links and corresponding rod end, teeter stop split in half and impact damage from mast bumping.
Source: ATSB and the United Kingdom Air Accidents Investigation Branch
Fuselage and cabin
Both windshields had shattered, however, the bow (central pillar) was intact. The left seat quick‑disconnect dual flight controls were installed. It was reported both doors were fitted when the helicopter departed Koorda.[24] The left door or its components were not identified in the wreckage at the site, nor in an extensive search of the surrounding area. It was possible it may have been destroyed during the main rotor blade strike to the cabin, but with the fragments unable to be identified or located in the loose muddy sand that was below the salt crust. There was no evidence of main rotor blade strike to any portion of the tailcone.
Post on-site examination
The governor controller was sent to the helicopter manufacturer in the United States for testing, observed by the ATSB (via video link). However, impact damage prevented any functional testing. An internal visual inspection revealed damage consistent with impact forces and there was no evidence of electrical arcing or overheating of the circuit board components.
The ATSB collected a small amount of fuel from the gascolator and auxiliary tank. This was tested by an independent accredited laboratory. While the fuel recovered had been contaminated with ground water after the collision with terrain, the fuel content was consistent with 100 LL Avgas.
Most of the lamps for the warning and caution lights, including low rotor RPM, low fuel and engine governor-off, had been destroyed by impact forces. The only lamps available for testing (clutch, alternator, main rotor chip and main rotor temperature) were examined at the ATSB’s technical facilities in Canberra, Australian Capital Territory. The main rotor chip and temperature lamp filaments had fractured and there was no observable stretch.[25] Examination of the alternator[26] and clutch[27] lamps indicated they probably were illuminated at impact. However, it was unknown if these indications would have illuminated prior to, during, or as a result of the impact sequence. Therefore, the status of these lights prior to the impact was unable to be determined.
In addition, examination of the instruments identified possible contact transfer of material from the needle to the instrument face, at 50-55% engine and 58% rotor RPM on the dual tachometer. The normal operating range for the engine was 101% to 104%, with a maximum continuous RPM of 104%. The engine RPM had to be maintained within this tolerance for the main rotor system to provide effective lift. A possible sweep mark at 11‑15 inches of mercury was identified on the manifold pressure gauge, which was lower than would be expected in cruise conditions. This could be indicative of severe icing, however, it was also possible that the sweep between 11 and 15 was from disruption due to impact forces. There were no reliable marks identified on the carburettor air temperature gauge face or internal components.
In early November 2022, the engine was disassembled and examined at a Civil Aviation Safety Authority (CASA) authorised engine overhaul facility under the supervision of the ATSB. The engine condition was consistent with the engine’s recorded time-in-service since overhaul. No internal or external damage was identified that may have prevented the engine from operating normally prior to the accident. No defects were identified in the induction system components, core engine, or cylinder assemblies that may have affected its pre-accident operation. One magneto was operationally tested with positive results, however, the other could not be tested due to impact damage. Both magnetos were also internally examined, and resistance tested with nil defects identified. Further, the carburettor was bench tested and internally examined, with no issues identified.
In summary, examination of the helicopter’s flight and engine control systems, drive train and powerplant did not indicate any pre-existing defects that may have affected the control or normal operation of the helicopter.
Medical and pathological information
Post-mortem examinations
A full post-mortem examination was performed on the pilot and a limited examination conducted on the passenger.[28] Within the limits of this examination, the pilot’s post‑mortem did not identify any significant natural disease.
The post-mortems identified multiple fractures and injuries to both the pilot’s and passenger’s arms, hands, and legs. Research has previously been conducted into injuries sustained by control seat occupants during an accident and the extent to which those injures would be consistent with the occupant of the seat having their hands or feet on the controls. However, some research has found that the passenger and pilot may exhibit similar injuries if the passenger grasps a solid structure, such as bracing in anticipation of an impact (Cullen, 2004; Gradwell and Rainford, 2016). In addition, the inverted impact with terrain and limited protection provided by the lightweight airframe could have also contributed to the nature of the injuries sustained. Therefore, the evidence was inconclusive in determining whether the pilot or the passenger was manipulating the flight controls. Further, it could not be established if either had a medical event that prevented the other from being able to maintain control of the helicopter.
Toxicology testing conducted on pilot
Toxicology testing conducted as part of the pilot’s post-mortem examination detected the presence of paracetamol, propranolol, and quinidine/quinine. Carbon monoxide detected in the samples was less than 5%.[29]
Paracetamol is a commonly used over the counter analgesic, but generally without adverse side effects significant for its use in aviation. Propranolol, quinine and quinidine are prescription medications with some potential side effects and interactions.
A review of records from the Pharmaceutical Benefits Scheme, from June 2018, indicated that the pilot held prescriptions and a dispensing history for propranolol and temazepam, but none for either quinidine or quinine.
Quinidine and its naturally occurring stereoisomer (mirror image) quinine are natural alkaloids[30] and are individually synthesised for pharmaceutical and medical purposes. The toxicology testing technique could not differentiate between the 2 compounds. Quinidine was typically used to treat heart arrythmias and quinine, as a pharmaceutical medication, to treat malaria. Quinine is also used as a bittering agent in soft drinks such as bitter lemon or tonic water. The pilot used tonic water as a mixer with alcoholic drinks but was reported to have not consumed any during the days prior to the accident.
Propranolol is a prescription medication in the Beta-blocker class of drugs, that blocks the release of stress hormones, such as adrenaline. It can be prescribed for a variety of conditions that include migraines, benign heart palpitations and performance anxiety. The use of propranolol in an aeromedical context is discussed below (refer to section titled Civil Aviation Safety Authority review of propranolol use).
Consultant pharmacologist review
The ATSB engaged a consultant pharmacologist to review the results from the pilot’s toxicology testing. That review found the level of propranolol detected was unlikely to have been consumed during the period 24-hours prior to the accident, nor did they anticipate any adverse effects on the pilot’s ability to operate the helicopter during the accident flight.
They also concluded that if the quinine or quinidine source was from tonic water (ingested as quinine), it would have most likely have had to be consumed within the previous 24 hours and at the detected levels, they would not have expected any impairment of the pilot’s performance. If the source was quinidine (as a medication), it could have been consumed during the previous few days.
Further, the consultant pharmacologist found that the carbon monoxide levels (less than 5% saturation) in the non-preserved blood of the pilot was insignificant.
Civil Aviation Safety Authority review of propranolol use
Although propranolol was not a medication prohibited for use by pilots, the reason for its use could be of aeromedical significance and for that reason, required assessment by a designated aviation medical examiner (DAME) and/or CASA. Aeromedical impacts for its use included the blocking of the effect of adrenaline in maintaining blood pressure under g‑loading and to also reduce blood pressure at normal g-loadings but making the pilot more prone to light headedness from other causes such as dehydration.
Due to the potential side effects, CASA advised the ATSB that propranolol would not usually be approved for pilots who were likely to encounter high g-loadings[31] (high performance aircraft) or operations involving complex variations in g-loadings (push-pull effects in particular). In other cases, pilots may be approved to use propranolol if they could demonstrate their blood pressure was consistently stable.
Pilots intermittently using propranolol to treat symptoms such as performance anxiety are required to be assessed for other effects (such as low blood pressure and tiredness) and could be advised to not operate an aircraft within 24 hours of taking propranolol.
Additional medical considerations
The pilot had previously been diagnosed by their general practitioner as having a longstanding familial benign essential tremor, affecting their hand’s fine motor skills. To treat those symptoms, propranolol had been prescribed and was to be taken as needed. That tremor had also been noticed by many of the pilot’s flying friends and colleagues and was reported to be particularly evident when using hand tools such as screwdrivers, writing and pouring drinks. Flight examiners, instructors and passengers who had flown with the pilot advised that, although they were aware of the existence of the pilot’s tremor, they had not noticed it to affect the operation of the helicopter or aeroplanes being flown.
The pilot completed an aviation medical with a CASA DAME every 2 years. That process required submission of an online applicant medical history questionnaire and then completion of a physical examination and tests with a DAME to assess the applicant’s ability to meet the relevant medical standard. This included assessing the applicant’s hand/eye coordination and checking for any symptoms or indications of neurological disease.
The DAME had conducted the pilot’s last 3 medical renewals, with the last being conducted in January 2022, and did not recall the applicant having any tremor of clinical significance. Further, the pilot had not declared in their applicant medical history questionnaire they had symptoms or were receiving treatment of any movement disorder (including tremor), or that they had been prescribed any medications. Had a tremor of clinical significance been identified, the DAME reported that they would have referred the pilot to a neurologist for a specialist opinion.
Extreme teeter and mast bumping
Under certain specific flight conditions, semi-rigid rotor systems are susceptible to extreme teetering where the blades teeter beyond their normal operational range, resulting in what is commonly known as ‘mast bumping’. Mast bumping is the act of the inboard end of the blade (the spindle) or main rotor hub contacting the main rotor shaft. In R22 helicopters, this can generally be identified by extensive damage to the teeter stops and varying degrees of structural damage to, and possible fracture of, the mast.
As documented in many investigation reports worldwide, scenarios that have been linked to mast bumping include low-g and/or low rotor RPM/rotor stall, in conjunction with delayed and/or inappropriate flight control inputs.[32]
The low-g condition
‘G’ or ‘g’ is an abbreviation for the acceleration due to the earth’s gravity. Positive ‘g’ is necessary for helicopters to respond to pilot control inputs. In a low-g condition (that is, approaching the feeling of weightlessness),[33],[34] the pilot’s ability to control the attitude of the helicopter is greatly reduced.
The United States Federal Aviation Administration (FAA) Helicopter Flying Handbook further described low-g effects, including:
Helicopters rely on positive G to provide much or all of their response to pilot control inputs. The pilot uses the cyclic to tilt the rotor disk, and, at one G, the rotor is producing thrust equal to aircraft weight. The tilting of the thrust vector provides a moment about the center of gravity to pitch or roll the fuselage. In a low-G condition, the thrust and consequently the control authority are greatly reduced.
Although their control ability is reduced, multi-bladed (three or more blades) helicopters can generate some moment about the fuselage independent of thrust due to the rotor hub design with the blade attachment offset from the center of rotation. However, helicopters with two-bladed teetering rotors rely entirely on the tilt of the thrust vector for control. Therefore, low-G conditions can be catastrophic for two-bladed helicopters.
… (when the helicopter) enters a low-G condition. Thrust is reduced, and the pilot has lost control of fuselage attitude but may not immediately realize it. Tail rotor thrust or other aerodynamic factors will often induce a roll. The pilot still has control of the rotor disk, and may instinctively try to correct the roll, but the fuselage does not respond due to the lack of thrust. If the fuselage is rolling right, and the pilot puts in left cyclic to correct, the combination of fuselage angle to the right and rotor disk angle to the left becomes quite large and may exceed the clearances built into the rotor hub. This results in the hub contacting the rotor mast, which is known as mast bumping.
RHC stated that extreme teetering and subsequent mast bumping can result from the pilot attempting to recover from an uncommanded right roll while in a low-g condition. Extensive investigation has shown that inappropriate recovery flight control inputs often resulted in the main rotor blade impacting the forward fuselage.
Low rotor RPM and stall
The R22 helicopter, with its low rotor system mass and relatively high RPM, is described as ‘low inertia’. In low inertia systems, rotor RPM is gained and lost very easily. Low rotor RPM occurs when the rotor can no longer produce enough lift to support the weight of the helicopter, and it will start to descend. If this situation is not quickly and effectively managed, the rotor RPM can reduce to a point where one, or both, main rotor blade/s stall. According to RHC safety notice[35] SN-24 Low RPM rotor stall can be fatal, rotor stall recovery is ‘virtually impossible’.
Low-rotor RPM can occur at almost any time, during power-on and power-off operations and is usually the result of improperly coordinating the collective and throttle, including overpitching or a failure to quickly lower the collective in an emergency such as engine failure or power reduction.
RHC safety notice SN-10 Fatal accidents caused by low rotor RPM rotor stall included:
A primary cause of fatal accidents in light helicopters is failure to maintain rotor RPM. To avoid this, every pilot must have his reflexes conditioned so he will instantly add throttle and lower collective to maintain RPM in any emergency.
…
Power available from the engine is directly proportional to RPM. If the RPM drops 10%, there is 10% less power. With less power, the helicopter will start to settle, and if the collective is raised to stop it from settling, the RPM will be pulled down even lower, causing the ship to settle even faster. If the pilot not only fails to lower collective, but instead pulls up on the collective to keep the ship from going down, the rotor will stall almost immediately. When it stalls, the blades will either "blow back" and cut off the tailcone or it will just stop flying, allowing the helicopter to fall at an extreme rate. In either case, the resulting crash is likely to be fatal.
No matter what causes the low rotor RPM, the pilot must first roll on throttle and lower the collective simultaneously to recover RPM before investigating the problem. It must be a conditioned reflex. In forward flight, applying aft cyclic to bleed off airspeed will also help recover lost RPM.
The low rotor RPM warning lamp and horn will activate when the rotor RPM reduces to 97% or below. The warning lamp is located on the top of the instrument panel and the horn can be heard in the cabin and in both headsets. The POH stated that ‘catastrophic rotor stall could occur if the rotor RPM ever drops below 80% plus 1% per 1,000 ft of altitude’. Further, the FAA Helicopter Flying Handbook stated that ‘low inertia rotor systems can become unrecoverable in 2 seconds or less if the RPM is not regained immediately’.
Potential factors leading to low-g and/or low rotor RPM/rotor stall
As a possible explanation for delayed or inappropriate control inputs that may have preceded a low-g condition or low rotor RPM and stall, the investigation explored the following scenarios:
Low-g pushover
RHC safety notice SN-11 Low-g pushovers – extremely dangerous stated ‘pushing the cyclic forward following a pull-up or rapid climb, or even from level flight, produces a low-g (weightless) condition’. It also stated that severe in-flight mast bumping usually results in main rotor shaft separation and/or rotor blade contact with the fuselage. The following warning was included:
Never attempt to demonstrate or experiment with low-G manoeuvres, regardless of your skill or experience level. Even highly experienced test pilots have been killed investigating the low-G flight condition. Always use great care to avoid any manoeuvre which could result in a low-G condition. Low-G mast bumping accidents are almost always fatal.
The ATSB spoke with the pilot’s regular helicopter flight instructor and other pilots who routinely flew with the pilot in VH-RAS. They all reported that the pilot demonstrated awareness of low-g flight conditions and the danger in entering a pushover manoeuvre, whether deliberate or unintentional. It was therefore determined to be unlikely that the pilot initiated a deliberate low-g pushover.
Turbulence
The FAA Helicopter Flying Handbook stated that ‘turbulence, especially severe downdrafts, can also cause a low-g condition and, when combined with high airspeed, may lead to mast bumping’. RHC safety notice SN-32 referred to flying in high winds or turbulence,[36] firstly stating that it should be avoided. It continued, noting that ‘a pilot’s improper application of control inputs in response to turbulence can increase the likelihood of a mast bumping accident’. In addition, in March 2024, the ATSB published the safety advisory notice Anticipate turbulence and slow down, which included:
Awareness of conditions likely to produce turbulence, and slowing down prior to encountering turbulence, could increase the time available to recognise and respond to a low-g condition in Robinson Helicopters.
Several pilots who departed Koorda that morning reported encountering some turbulence over the Cowcowing Lakes area.[37] RHC recommended a reduction in airspeed when encountering turbulence. The recorded data for VH-RAS did not indicate any significant deviation to altitude or airspeed typically seen in moderate, severe or extreme turbulence. In addition, the data did not indicate any significant reduction in airspeed in the last minutes of the flight, as recommended by RHC when encountering significant turbulence.[38],[39]
RHC also advised helicopters that are lightly loaded may be more susceptible to turbulence than heavy helicopters. As the weight of VH-RAS was calculated to be at, or just above, its maximum weight at the time of the accident, this potentially reduced any effects of turbulence that may have been encountered.
Overpitching
As the collective is raised, there is a simultaneous and equal increase in pitch angle of both main rotor blades. An increase in pitch angle also results in increased drag on the main rotor blades. To counter this adverse effect, the R22 has a throttle correlator mechanism attached to the collective control that increases the engine’s throttle when the collective is raised.
Overpitching is a condition that happens when the collective pitch is increased to a point where the angle of attack of the main rotor blades creates extra drag and maximum engine power cannot maintain or restore normal operation rotor RPM. One typical scenario often occurs during take-off, where the pilot raises the collective lever to a point beyond the full throttle position (where full throttle was required to maintain RPM), then there will be more power required by the rotors than power available from the engine, resulting in a rotor RPM decay. However, as the helicopter had departed Koorda without incident, this was considered unlikely for this occurrence.
Overpitching can also occur if the pilot raises the collective lever at a rate that is faster than the correlator will open the throttle (to avoid a bird for example), while not compensating for the increased drag by manually increasing the throttle. In this case, the rotor RPM may rapidly decay to a level that is too low for the engine power available to recover.
In addition, overpitching is more likely to occur at a high weight and/or high altitude, where the rotor blades are already operating at larger pitch angles.
Any application of collective to arrest the descent further increases rotor drag and reduces rotor RPM. The situation can rapidly deteriorate resulting in the rotor blades effectively stalling and significantly reduced lift.
Potential for negative transfer of learning in an emergency situation
The pilot had considerable aeroplane operational experience, including flight activity endorsements to conduct aerobatics, spinning and formation flying. The investigation considered if the pilot may have reacted to an unexpected situation with an inadvertent ‘aeroplane’ control input, as described in SN-29 Airline pilots high risk when flying helicopters, which could have induced a low-g scenario. Specifically, the notice stated that:
…The airplane pilot may fly the helicopter well when doing normal maneuvers under ordinary conditions when there is time to think about the proper control response. But when required to react suddenly under unexpected circumstances, he may revert to his airplane reactions and commit a fatal error. Under those conditions, his hands and feet move purely by reaction without conscious thought. Those reactions may well be based on his greater experience, ie., the reactions developed flying airplanes.
Records indicated the majority of the pilot’s recent flying had been conducted in VH-RAS.[40] In addition, people who had flown in VH-RAS reported the pilot to being cognisant of the dangers of inappropriate control inputs when operating an R22 helicopter. Therefore, while a scenario where the pilot inadvertently used aeroplane flight control actions was considered a possibility, it was assessed to be unlikely in this occurrence given the pilot’s helicopter experience.
Inadvertent or inappropriate dual control input
Several sources reported that, on occasion, the pilot had offered passengers with flying experience the opportunity to ‘fly’ the helicopter using the left seat dual flight controls. Some passengers reported that they had flown the helicopter using the cyclic control, while the pilot retained control of the collective. One of those passengers had remarked that the pilot had been closely supervising them and had cautioned them about making abrupt forward control inputs. Another passenger recalled that they had been offered but decided to decline the opportunity to fly the helicopter. In this case, the pilot had emphasised the sensitivity of the flight controls and highlighted the hazards with making abrupt control inputs.
RHC safety notice SN-20 Beware of demonstration or initial training flights noted that, ‘a disproportionate number of fatal and non-fatal accidents occur during demonstration or initial training flights’. Further, if a ‘student’ was to make sudden large control movements in the wrong direction, an experienced instructor may not necessarily be able to recover control of the helicopter. The notice also highlighted the importance of thoroughly explaining to students the ‘extreme sensitivity of the controls in a light helicopter’.
RHC safety notice SN-44 Carrying passengers also identified that carrying a passenger can potentially increase risk as they add workload and distraction. The notice specifically stated:
- Always remove passenger-side controls.
- Caution passengers against inadvertently bumping the cyclic center post.
Another potential source of confusion for someone inexperienced in flying helicopters is the throttle operation. The throttle twist control, located on the end of each collective, is operated by rolling the hand away from the body (or outboard) to increase engine power, and rolling toward the body to decrease. This is opposite to throttle controls fitted to vehicles such as motorcycles and the steering arm on boat outboard motors.
Despite the above, without any onboard recording devices such as a cockpit camera, the ATSB was unable to determine if the passenger was in control or if they had inadvertently bumped or moved the controls.
Other scenarios
The investigation considered other scenarios that may induce a large control input, such as avoiding a bird or a door opening or separating in-flight. Bird avoidance may have resulted in an abrupt control movement, however, nil evidence of a strike was found in and around the wreckage. Further, one of the pilots in the group and a local resident stated that they did not observe any bird activity in the area.
If a door had opened, this had potential to temporarily distract the pilot and/or the passenger, or result in an inadvertent control input while trying to close the door. [41] Noting that the left door could not be identified at the accident site, operating the helicopter with one or both doors removed is permitted, with the manufacturer advising to calculate weight and balance as required[42] and ensure loose articles are secured in the cabin. Liberation of the left door had the potential to strike the tail cone or tail rotor assembly, however, there was no evidence of the door coming into contact with any portion of the tailcone.
While these scenarios remained a possibility, there was insufficient evidence to conclude probability.
Engine power loss scenarios
While the governor switch was found in the OFF selection, the position prior to impact could not be determined and post-accident functionality testing could not be performed due to the damage sustained. However, it remained a possibility for an intermittent issue (for example a cylinder misfire, fouled spark plug or sticky valve), to briefly affect engine operation, which did not result in any visible damage. An intermittent loss of engine power will typically result in a ‘kick’ (nose left yaw). It was possible that a sudden yaw may have contributed to either the pilot or passenger making a large control input, but there was insufficient evidence to conclude probability.
There have also been cases where an engine stoppage was inadvertently induced by rolling off the throttle too fast or the mixture control being pulled instead of the carburettor heat or another control.[43]
Acknowledging the limitations of the Civil Aviation Safety Authority Carburettor icing probability chart and variations to induction systems depending on engine installation, the investigation also considered the possibility of carburettor icing. Carburettor ice is formed when the normal process of vaporising fuel in a carburettor cools the carburettor throat so much that ice forms from the moisture in the airflow, which can restrict airflow to the engine. This is more likely to occur at low engine power settings due to the added cooling effect of the partially-closed throttle butterfly.[44],[45] This valve provides more area on which the ice can accrete and increases the partial vacuum downstream of the valve. This causes further chilling of the air and the water droplets, further increasing the likelihood of ice accretion. The effect of carburettor icing includes reduced power output, rough running and in some cases engine failure. Further, the risk of ice build-up in the carburettor can be high even with no visible moisture and at temperatures of up to 38 °C.
The RHC safety notice SN-25 Carburetor Ice also stated that, ‘avoidable accidents have been attributed to engine stoppage due to carburetor ice. When used properly, the carburetor heat and carb heat assist systems on the R22 and R44 will prevent carburetor ice’. The European Union Aviation Safety Agency safety information bulletin No: 2010-03 Carburetor Icing Prevention, also noted that:
In a helicopter, icing can develop quite insidiously and the effect on the engine is less obvious. Furthermore, should the engine stop, an immediate entry to autorotation is necessary to prevent catastrophic reduction of rotor RPM, and the descent rate, usually around 2 000 feet per minute, is such that there is rarely time to attempt a restart.
RHC safety notice SN-31 Governor can mask carb ice stated that, when ice begins to form in the carburettor, a properly functioning governor will increase throttle to maintain engine RPM, which will also result in constant manifold pressure. Once the governor has opened the throttle completely it can no longer maintain rotor RPM and the engine RPM will reduce, which may be the first point a pilot is aware of the icing, if they had not been monitoring the manifold pressure and carburettor air temperature gauges.[46],[47] Further, application of heat at this late stage will melt any ice, which could result in engine stoppage.
The environmental conditions and time between the accident and the ATSB’s examination of the wreckage meant that any icing in the throat of the carburettor would have melted and not been detectable. Also, the examination could not determine if carburettor heat was in use prior to impact nor were there any reliable needle impact marks identified on the carburettor air temperature gauge. Therefore, while local conditions were conducive to the possibility of the formation of carburettor ice, it was not possible to establish if VH-RAS was affected by carburettor icing at any stage of the flight.
Overall, the ATSB’s examination of the engine did not identify any issue that may have affected operation and noting that the engine was likely rotating at impact, may suggest that an engine stoppage had not occurred. However, the investigation could not conclude an intermittent engine issue and/or that carburettor icing occurred, resulting in a reduction in power and the requirement to enter autorotation. Equally, the inverted portion of the descent had the potential to interrupt fuel flow to the engine via the gravity‑fed fuel system and reduce engine power.
In the event of an engine power failure above 500 ft above ground level, the emergency procedures section of the POH stated to:
- Lower collective immediately to maintain rotor RPM
- Establish a steady glide at approximately 65 KIAS (knots indicated airspeed)
- Adjust collective to keep RPM between 97 and 100% or apply full collective down if light weight prevents attaining above 97%.
Airspeed and descent rates may be variable during controlled descent. Descent rates during autorotation vary depending on helicopter configuration and local conditions, however, anywhere between 1,400 to 1,900 ft/min can be expected.[48]
However, using the recorded data, the investigation estimated that the helicopter collided with terrain between 10 to 15 seconds after departing from controlled flight. The descent rate of about 9,000 ft/min during this period was inconsistent with the helicopter being established in an autorotative descent.
Low-g or low rotor RPM signatures
The ATSB liaised with RHC and reviewed investigation reports from the United Kingdom, United States, Europe, and New Zealand. Excessive teeter and mast bumping, such as fractured tusks and teeter stops, were associated with a combination of these scenarios.
A sudden roll to the right is often associated with a low-g event. Typical low-g wreckage signatures include a main rotor blade strike to the cabin/forward fuselage (where the blade/s cuts through the helicopter at high velocity) and mast separation (often at the mast bump/teeter stop location). In addition, a long/large debris trail is indicative of in‑flight break‑up initiating when the helicopter was within normal operating parameters.
Low rotor RPM and rotor stall signatures identified in many investigation reports included a rotor strike to the tailcone, main rotor blade coning,[49] spindle impact marks on the main rotor head, little to no rotational ground marks, and the helicopter nosing over and a vertical descent with a small debris field. However, it has been documented that, since RHC changed the main rotor blade construction from stainless steel skin to aluminium skin, damage to blade skins due to extreme coning as a result of low rotor RPM is now less than previously observed.[50]
A rotor strike to a solid object, such as the fuselage, a tree, or the ground, can stop a low powered engine. This is in contrast to where rotor blades often fracture into multiple small pieces during a rotor strike with full engine power.
Similar occurrences
A review of investigation reports from Australia, New Zealand, United Kingdom, United Sates, South Africa, and Europe identified at least 3 reports that had signatures very similar to this occurrence. These are summarised below.
German Federal Bureau of Aircraft Accident Investigation BFU21-0949-3X
On 17 October 2021, recorded data from a Robinson R44 Raven II showed that, while in cruise about 1,900 ft above mean sea level, the helicopter abruptly climbed about 200 ft, followed almost immediately by a rapid descent. Coincident to this, the recorded ground speed rapidly decayed from about 100 kt to zero. The pilot and 2 passengers were fatally injured.
The mast had fractured below the swashplate and the rotor head, with the blades attached, came to rest on top of the upright destroyed fuselage. Typical mast bump and in‑flight break‑up signatures were found on the mast and rotor head. One main rotor blade showed damage consistent with it striking the forward fuselage. In addition, the left seat (passenger) dual flight controls were installed.
The investigation concluded the helicopter likely entered a low-g condition that led to mast bumping ‘due to erroneous control inputs, which resulted in the rotor hitting the fuselage and in‑flight break-up’. Of note, the Bureau indicated that it was quite possible that the passenger was allowed to control the helicopter during the flight and unknowingly initiated a pull‑up or push over. However, it could not be established with any certainty who was in control of the helicopter at the time of the occurrence.
ATSB investigation AO-2020-061
On the afternoon of 2 December 2020, a RHC R44 Raven I, registered VH-HGU, departed Goulburn Airport, New South Wales with a student pilot and instructor on board. The helicopter flew east, and the last recorded automatic dependent surveillance broadcast detected it descending into a valley in the Bungonia State Conservation Area. A search commenced when the helicopter did not return as expected, and the wreckage of VH-HGU was found in a valley, approximately 4 km north-west of its last ADS-B transmission. Both pilots were fatally injured, and the helicopter was destroyed.
Although fire and impact damage had destroyed some parts of the helicopter, the evidence available gave no indication that the helicopter was operating abnormally prior to an in‑flight break-up. Components recovered near the beginning of the 275 m wreckage trail indicated that a main rotor blade had struck the left side of the fuselage at the beginning of the break‑up sequence. No evidence was found to indicate pre-existing mechanical defects or issues that could have prevented normal engine operation. While a transient condition such as a partial or complete power loss could not be ruled out, such an event should not have resulted in an in-flight break-up.
The investigation concluded that, while flying in the vicinity of the valley, the helicopter entered a low-g condition due to turbulence, inappropriate control inputs, or a combination of both. This condition, probably in combination with inappropriate recovery control inputs resulted in extreme teetering of the main rotor. A mast bump occurred as a result, and the helicopter subsequently broke up in-flight.
United Kingdom Air Accidents Investigation Branch G‑CHZN
On 6 January 2012, after about 1 hr and 28 minutes into a private flight, and when about 1,440 ft above the ground, the Robinson R22 disappeared from air traffic control radar. Witnesses reported hearing a pop sound and some described the helicopter rolling to the left, with one person saying the helicopter pitched up prior to the left roll. The helicopter then fell, inverted, to the ground. The pilot, the only person on board, sustained fatal injuries.
Both main rotor blades had separated from the hub. There was evidence of rotor blade strike to the forward left fuselage. The tailcone remained attached to the fuselage and there was no evidence of rotor strike. An engine examination did not identify any issue or failure that would prevent it from operating normally. The local conditions at the time were calculated to be conducive to moderate or serious carburettor icing at any engine power.
The investigation explored low-g and low rotor RPM, with the wreckage exhibiting signatures from both scenarios. Pilot incapacitation, carburettor icing and avoiding a bird were also considered as possible contributing events. The report concluded that the mast bumping ‘was probably caused by a loss of rotor RPM (not followed by rapid lowering of the collective), a low-g pushover, a large abrupt control input – or a combination thereof’. Further, it was noted that low-g or a large abrupt control input ‘could have been generated for a number of reasons, and the light control forces in the R22 make it relatively easy to enter such conditions’.
Safety analysis
Introduction
On the morning of 2 October 2022, a Robinson Helicopter R22 Beta II, registered VH‑RAS, departed Koorda, Western Australia, on a return flight to Jandakot via Northam aerodrome. Shortly after reaching cruise, the helicopter departed from controlled flight, descended rapidly, and collided with terrain inverted. The 2 occupants were fatally injured, and the helicopter was destroyed.
This analysis will examine the factors that likely contributed to the in-flight break-up, which include entering a low-g and/or low rotor RPM/rotor stall condition. It will also discuss the fitment of dual flight controls when carrying passengers and the importance of disclosing to aviation medical specialists the use of prescription medication for a medical condition.
Departure from controlled flight due to in-flight break-up
Analysis of the recorded data indicated that the helicopter took off from Koorda and climbed to a cruise altitude with no apparent issues. However, shortly after, the data broadcast by the iPhone was consistent with the helicopter departing controlled flight. The groundspeed quickly reduced and the helicopter rapidly descended at a rate significantly in excess of what would be expected if an autorotation was being conducted by the pilot. The trajectory apparent in the analysis of the iPhone data closely correlated with the location of the helicopter wreckage.
Extreme teetering
The site and wreckage examination identified signatures consistent with the main rotor assembly being subject to excessive teeter and mast bumping. This included fracture of the spindle tusks, teeter stops, pitch links, and main rotor strike to the fuselage. While the main rotor assembly separation location was lower than the typical mast bumping, impact damage in the vicinity of the teeter stops was indicative of the severe forces associated with extreme teetering. In this instance, the mast fracture was likely associated with pitch horn impact, from a freely rotating blade, and determined to have occurred later in the accident sequence. As a result of the extreme teetering and mast bumping, the helicopter was subject to structural failure and in-flight break-up, beyond which sustained flight was no longer possible.
Low-g and/or low rotor RPM/stall conditions
The ATSB examined several previous accidents with similar circumstances, all of which identified low-g or low rotor RPM/rotor stall as conditions that could lead to extreme teetering and/or mast bumping and an in-flight break-up.
Examination of the dual tachometer identified signatures of low rotor and engine RPM at impact, however, this may not be indicative of operational conditions just prior to the departure from controlled flight. Although the small debris field and flight data showing an almost vertical descent were representative of low rotor RPM/rotor stall, there was only minor coning to the main rotor blades, but this may have been due to the aluminium skin construction of the main rotor blades. In addition, it was unknown if the low rotor RPM warning light was illuminated as the lamp was destroyed.
While the helicopter impacted the ground inverted, it could not be established if this was initiated by a right roll (low-g) or a nose over event (low rotor RPM). The method of the main rotor blade strike to the cabin was consistent with a mast bumping event and likely occurred during the later stage of the uncontrolled descent.
Multiple scenarios were determined to have preceded a low‑g or low rotor RPM condition, which were also explored by the ATSB. These included responding to an engine power loss/failure (such as from carburettor icing or governor unit malfunction), turbulence, low-g pushover, overpitching, a medical event, birdstrike or bird avoidance, a door opening or separating in-flight, or other large control input for undetermined reasons. While some of these were considered unlikely, as discussed previously, some were inconclusive due to insufficient evidence and the absence of an onboard camera.
However, the preceding factors examined result from, or could be exacerbated by, pilot flight control inputs. For example, inappropriate inputs may result in a low-g pushover or overpitching, incorrect recovery inputs when responding to turbulence may result in low-g, or delayed inputs when responding to a low rotor RPM may lead to rotor stall. In this instance, the ATSB was unable to establish which scenario preceded the other during the accident sequence, in that, pilot reaction to low-g may have inadvertently induced a low rotor RPM state, or vice versa.
Research has shown that, even if a helicopter enters a low-g or low rotor RPM condition it is recoverable through prompt and appropriate control inputs. The RHC POH and safety notices provide detailed information on how to avoid these conditions, and best practice for recovering control if required. It is important to acknowledge that the automation of some systems (for example, the carburettor heat assist and engine RPM governor) may mask a developing malfunction or adverse condition. While flight training assists in appropriate recovery techniques becoming instinctual, the pilot must remain vigilant throughout the entire flight to avoid a delayed reaction in an unexpected situation. This is critical as the R22 low inertia rotor system means a pilot could have less than 2 seconds to identify the situation and react appropriately before it becomes unrecoverable.
In summary, the site and wreckage examination identified signatures that were likely indicative of a low-g and/or low rotor RPM/rotor stall condition. While an onboard camera was not fitted, given the nature of the events that typically precede these conditions, it was also likely that delayed and/or inappropriate flight control inputs were a factor, although the exact circumstances could not be conclusively determined. However, it is known that low‑g and low rotor RPM/rotor stall conditions can be catastrophic for helicopters with semi-rigid rotor heads. Therefore, a pilot’s ability to identify the condition and promptly apply the correct flight control inputs is vital to effective recovery and continued safe operation.
Dual flight controls
Examination of the wreckage identified that the quick-disconnect dual flight controls were installed at the left seat (passenger) location. It was also established that, on occasion, the pilot allowed passengers with previous flying experience to operate the cyclic control. As large, abrupt control inputs are one precursor to teetering/mast bumping events, the ATSB considered the possibility of an inadvertent control input from the passenger, such as bumping the controls, or the passenger having control of the cyclic and/or any of the other dual controls.
The cyclic T-bar ‘see-saw’ design meant that, if the person seated in the left seat has control of the helicopter, the cyclic grip of the right seat person would be in a higher-than-normal operating position. In a situation where a passenger has made an inappropriate control input from the left seat, the positioning of the T-bar could potentially delay the pilot from regaining control. RHC noted that, even within a structured flying training context, the instructor may not be able to regain control of the helicopter in time to prevent a loss of control.
Having dual flight controls installed increases the risk of inadvertent control inputs by a passenger. This is supported by the advice in the R22 POH to remove the controls and in a further safety notice from RHC, which described the risks when carrying passengers. While there was insufficient evidence in this case to determine if the passenger made an inadvertent control input or if they were operating any of the controls during the flight, either action had the potential to contribute to the helicopter entering a low-g and/or low rotor RPM condition.
In addition, to avoid an inappropriate input as much as possible, unless operating in a flight training environment with a qualified instructor, under no circumstances should an unqualified person be permitted to manipulate the helicopter’s controls. As emphasised in the RHC safety notice on demonstration or initial training flights, this is particularly relevant in the R22 type helicopter, where the low inertia rotor system affords a pilot very little time to react appropriately and regain control.
Disclosure of medical information
While a medical event prior to the in-flight break-up could not be determined in the absence of an onboard camera, the investigation established that the pilot had been prescribed and was taking medication (propranolol) to treat symptoms of their hand tremor. However, this information had not been declared to the DAME during their recent medical examinations. This precluded an opportunity by CASA and/or the DAME to complete a formal assessment of that condition and the use of medication for aeromedical significance and fully assess the pilot’s ability to meet the relevant medical standard.
Based on information provided to the ATSB by CASA and the DAME who had completed the pilot’s most recent medical renewals, neither the hand tremor nor the prescription medication used to treat those symptoms would necessarily have precluded the pilot being issued an aviation medical certificate.
Although the source of the quinine or quinidine could not be determined, the level of this and the propranolol detected were unlikely to have affected the pilot’s ability to operate the helicopter, as assessed by the consultant pharmacologist. Similarly, those who had flown with the pilot stated that they did not recall the tremor affecting their ability to fly.
Nevertheless, it is important to declare all medications and medical conditions to address risks that could affect performance. While it is acknowledged that some pilots may have concerns about not meeting medical certificate requirements if they make such declarations, pathways exist for managing certain medical conditions while maintaining a medical certificate.
Cockpit cameras
The ATSB explored multiple scenarios that were considered to contribute to extreme teetering and mast bumping accidents. However, there was insufficient evidence available to determine the events immediately prior to the in-flight break-up. As noted by the Transport Accident Investigation Commission (2021), a significant proportion of mast bumping accidents in New Zealand have occurred in low-g flight conditions. However, ‘Part of the problem is that the available evidence has not allowed the circumstances and causes of all of these ‘mast bumping’ accidents to be fully determined’. As such, the Commission recommended the need for cockpit video recorders and/or other means to capture data in certain classes of helicopter.
In recent years, RHC has introduced cockpit cameras into the R66 and R44 helicopters as standard. The inclusion of these cameras will provide vital footage and audio information to investigators and manufacturers. Understanding the circumstances leading up to extreme teeter and in-flight break events, will assist in determining appropriate steps for ongoing safety improvement.
Findings
ATSB investigation report findings focus on safety factors (that is, events and conditions that increase risk). Safety factors include ‘contributing factors’ and ‘other factors that increased risk’ (that is, factors that did not meet the definition of a contributing factor for this occurrence but were still considered important to include in the report for the purpose of increasing awareness and enhancing safety). In addition ‘other findings’ may be included to provide important information about topics other than safety factors. These findings should not be read as apportioning blame or liability to any particular organisation or individual. |
From the evidence available, the following findings are made with respect to the in-flight break-up involving Robinson R22 Beta II, VH-RAS, 13 km south-west of Koorda, Western Australia, on 2 October 2022.
Contributing factors
Other factors that increased risk
- Quick-disconnect dual flight controls were installed in a position occupied by a passenger, which increased the risk of inadvertent or inappropriate passenger control input.
- The pilot did not disclose their use of a prescription medication being used to treat symptoms of a medical condition to the Civil Aviation Safety Authority. This precluded specialist consideration and management of the on-going flight safety risk the medical condition and medication may have posed.
Other findings
- In helicopters with semi-rigid rotor heads, the circumstances leading to an in-flight break-up as a result of mast bumping and extreme teetering are not well documented. Recorded cockpit imagery would provide valuable information and insight into the events leading up to this type of occurrence.
Sources and submissions
Sources of information
The sources of information during the investigation included the:
- Civil Aviation Safety Authority
- Western Australia Police Force and the Coroner’s Court of Western Australia
- Robinson Helicopter Company
- maintenance organisation for VH-RAS
- Bureau of Meteorology
- participants of the flying event
- pilot’s flight instructor
- people who had regularly flown with the pilot
- consultant pharmacologist
- recorded data from a handheld GPS receiver and OzRunways computer server.
References
Gradwell, D. & Rainford, D.J. (Eds.) (2016). Ernsting’s Aviation and Space Medicine (5th ed). Boca Raton, FL: CRC Press.
Mornington-Sanford, R. (2014). It’s all in your head. Retrieved from https://www.morningtonsanfordaviation.com/
Mornington-Sanford, R. (2012). No ice, thank you. Retrieved from https://www.morningtonsanfordaviation.com/
Rivera, J., Talone, A.B., Boesser, C.T., Jentsch, F. & Yeh, M. (2014). Startle and surprise on the flight deck: Similarities, differences, and prevalence. In Proceedings of the human factors and ergonomics society annual meeting September 2014 (Vol. 58, No. 1, pp. 1047-1051). Sage CA: Los Angeles, CA: SAGE Publications.
Transport Accident Investigation Commission. (2021). Robinson helicopters: mast bumping accidents in NZ. Retrieved from https://www.taic.org.nz/watchlist/robinson-helicopters-mast-bumping-accidents-nz
Submissions
Under section 26 of the Transport Safety Investigation Act 2003, the ATSB may provide a draft report, on a confidential basis, to any person whom the ATSB considers appropriate. That section allows a person receiving a draft report to make submissions to the ATSB about the draft report.
A draft of this report was provided to the following directly involved parties:
- the maintenance organisation for VH-RAS
- Civil Aviation Safety Authority
- United States National Transportation Safety Board
- Robinson Helicopter Company
- Bureau of Meteorology.
Submissions were received from the Robinson Helicopter Company. The submissions were reviewed and, where considered appropriate, the text of the report was amended accordingly.
Appendices
Appendix A: Handheld GPS and iPhone data during accident flight
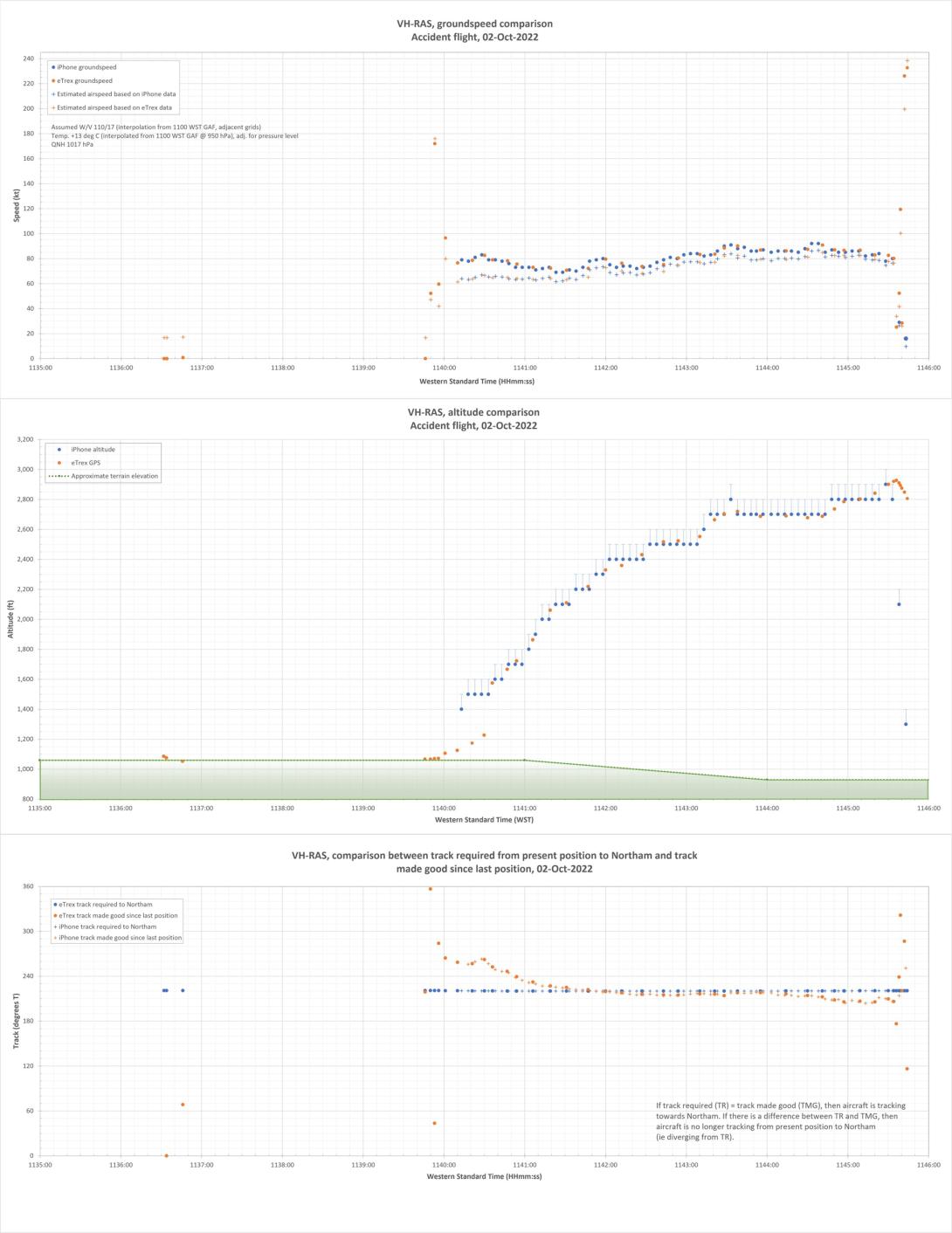
Purpose of safety investigationsThe objective of a safety investigation is to enhance transport safety. This is done through:
It is not a function of the ATSB to apportion blame or provide a means for determining liability. At the same time, an investigation report must include factual material of sufficient weight to support the analysis and findings. At all times the ATSB endeavours to balance the use of material that could imply adverse comment with the need to properly explain what happened, and why, in a fair and unbiased manner. The ATSB does not investigate for the purpose of taking administrative, regulatory or criminal action. TerminologyAn explanation of terminology used in ATSB investigation reports is available here. This includes terms such as occurrence, contributing factor, other factor that increased risk, and safety issue. Publishing informationReleased in accordance with section 25 of the Transport Safety Investigation Act 2003 Published by: Australian Transport Safety Bureau © Commonwealth of Australia 2024 ![]() Ownership of intellectual property rights in this publication Unless otherwise noted, copyright (and any other intellectual property rights, if any) in this report publication is owned by the Commonwealth of Australia. Creative Commons licence With the exception of the Coat of Arms, ATSB logo, and photos and graphics in which a third party holds copyright, this publication is licensed under a Creative Commons Attribution 3.0 Australia licence. Creative Commons Attribution 3.0 Australia Licence is a standard form licence agreement that allows you to copy, distribute, transmit and adapt this publication provided that you attribute the work. The ATSB’s preference is that you attribute this publication (and any material sourced from it) using the following wording: Source: Australian Transport Safety Bureau Copyright in material obtained from other agencies, private individuals or organisations, belongs to those agencies, individuals or organisations. Where you wish to use their material, you will need to contact them directly. |
[1] Visual flight rules (VFR): a set of regulations that permit a pilot to operate an aircraft only in weather conditions generally clear enough to allow the pilot to see where the aircraft is going.
[2] Avgas: a type of aviation fuel used in aircraft with a spark-ignited internal combustion engine.
[3] Time-in-service recorded on the maintenance release was collective activated. Therefore, engine running time (warm up, cool down and any time with the helicopter running on the ground) was not included.
[4] VH-RAS was the second R22 helicopter that the pilot had owned. Ownership of these helicopters was reported to have been transferred in 2016, as an operational consideration. Although VH-RAS had accumulated a higher number of hours total time in service prior to the ownership transfer, it had adequate hours available for the pilot’s purposes and their pattern of personal use.
[5] The 45 minutes included engine running time while the helicopter was on the ground, which resulted in about 20 minutes recorded on the maintenance release.
[6] Autorotation is a condition of descending flight where, following engine failure or deliberate disengagement, the rotor blades are driven solely by aerodynamic forces resulting from rate of descent airflow through the rotor. The rate of descent is determined mainly by airspeed.
[7] Coning of main rotor blades: the upwards movement of the main rotor blades while they are rotating. This is usually in response to an increase in aerodynamic force as a result of a control input from the pilot. It is more pronounced at high weights and/or low main rotor speed.
[8] The swashplate consists of 2 main parts: a stationary swashplate and a rotating swashplate. The stationary (inner) swashplate is mounted on the main rotor mast and is connected to the cyclic and collective controls by the push-pull tubes. It is able to tilt in all directions and move vertically. The rotating (outer) swashplate is mounted to the stationary swashplate by means of a bearing, which allows it to rotate with the mast. The swashplates move as one unit. The rotating swashplate is connected to the main rotor blade pitch horns by the pitch links.
[9] Cyclic: a primary helicopter flight control that is similar to an aircraft control column. Cyclic input tilts the main rotor disc, varying the attitude of the helicopter and hence the lateral direction.
[10] Quick-disconnect flight controls do not require use of tooling to fit and remove and can therefore be accomplished by the pilot, without the requirement for a licenced aircraft maintenance engineer.
[11] The POH required the pilot to use carburettor heat as required to keep the needle on the carburettor air temperature gauge out of the yellow arc (-15 to 5°C). In addition, carburettor heat was to be used with power settings below 18” mercury, regardless of the indicated carburettor air temperature.
[12] Visual meteorological conditions: an aviation flight category in which visual flight rules flight is permitted – that is, conditions in which pilots have sufficient visibility to fly the aircraft while maintaining visual separation from terrain and other aircraft.
[13] SIGMET provides information on the occurrence or expected occurrence of enroute weather phenomena that are potentially hazardous to aircraft.
[14] AIRMET provides information on deteriorating conditions, not already included in the relevant graphical area forecast.
[15] Cloud cover: in aviation, cloud cover is reported using words that denote the extent of the cover – ‘scattered’ indicates that cloud is covering between about 3/8 and half of the sky.
[16] Dewpoint: the temperature at which water vapour in the air starts to condense as the air cools. It is used, among other things, to predict the probability of aircraft carburettor icing or the likelihood of fog.
[17] Light turbulence results in momentary slight and erratic changes in attitude and/or altitude with little effect on loose objects. Moderate turbulence results in appreciable changes in attitude and/or altitude but the pilot remains in control at all times, Unsecured will objects move and there is an appreciable strain on seatbelts. Severe turbulence results in large abrupt changes in attitude and/or altitude and a momentary loss of control. Unsecured objects are tossed about and the occupants are violently forced against seatbelts (Bureau of Meteorology).
[18] OzRunways is an electronic flight bag application, utilising approved data for electronic maps and charts, and can be used to assist with navigation.
[19] The Australian Maritime Safety Authority’s Joint Rescue Coordination Centre had used this data during their initial response to the reports of the missing helicopter, which assisted with its prompt location.
[20] Taking into account the recorded groundspeed, the forecast wind, atmospheric pressure and temperature were used to estimate the calibrated airspeed (CAS) of the helicopter during the climb.
[21] The intended path of the aircraft over the ground, from the current position to reach the next waypoint or destination.
[22] The aircraft’s actual track over the ground.
[23] ‘SCEET’ is a high-temperature, flexible type aircraft ducting, constructed of 2 plies of silicone rubber impregnated fiberglass, supported with wire between the plies.
[24] The passenger on the flight from Jandakot to Koorda advised the ATSB that both doors were fitted for that flight. In addition, no one reported seeing either door being removed at Koorda.
[25] Filament stretching can be indicative of a hot filament and therefore the light being illuminated at impact.
[26] The alternator lamp will illuminate to indicate low voltage, typically alternator failure or when the engine was at or below idle speed of about 55% (1,512 rpm).
[27] The clutch lamp illuminates to indicate actuator operation and belt tensioning, which can occur during flight as the drive belts warm and stretch. Clutch lamp illumination could have been a normal function, or from the actuator trying to keep the drive belts tensioned due to airframe distortion during the accident sequence.
[28] A full post-mortem includes a full external and internal examination. While the extent of a limited post-mortem examination can vary, an external examination is performed (https://www.pathwest.health.wa.gov.au/).
[29] Carbon monoxide is a colourless, odourless and tasteless poisonous gas. It is a byproduct of the incomplete combustion of carbon containing materials such as exhaust gases from aircraft engines.
[30] Alkaloids are nitrogenous organic compounds of plant origin that have pronounced physiological effects on humans.
[31] Load factor being experienced by the pilot/aircraft, in relation to the normal force of gravity.
[32] Although not directly related to this occurrence, RHC has developed a symmetrical horizontal stabilizer for the R22 model helicopters, which enhances roll stability during high-speed flight. It is expected to be in production by mid-2024 with a retrofit kit for existing helicopters available shortly after. This new design is currently available on the R44 and R66 models.
[33] All 2-bladed teetering main rotor systems helicopters, including Bell 205/UH-1 and Bell 206/L, are subject to mast bumping.
[34] More detailed information is available from the Robinson Helicopter Company Low-G Mast Bumping Research paper, published 26 October 2022. Additional mast bumping research is available via Robinson Reference Materials - Robinson Helicopter Company
[35] RHC safety notices mentioned in this report are included in the POH and also freely available via the website - https://robinsonheli.com/
[36] Atmospheric turbulent eddies occur in a range of scales from hundreds of kilometres down to centimetres. Aircraft bumpiness is most pronounced when eddies are about the size of the aircraft, i.e. in the order of one hundred metres or so for commercial aircraft, to tens of metres for smaller aircraft. The reactions of aircraft are dependent on their type, configuration and the speed at which they encounter turbulent zones. Refer www.bom.gov.au for more detail.
[37] Analysis of track data for this aircraft indicated it had passed about 1.8 km abeam (north-west) the accident site at an altitude of 4,500 ft, about 1 minute prior to the accident. Therefore, aircraft-induced turbulence was considered not to be a factor in this occurrence.
[38] RHC safety notice SN32 included: ‘what is considered significant turbulence will depend on pilot experience and comfort level’.
[39] ATSB’s analysis of the available data indicated a relatively small reduction in estimated airspeed during the final stages of the flight. However, it was not possible to establish if this was an intentional action on the part of the pilot in response to encountering unexpected turbulence, a normal variation in airspeed during normal flight or an issue/malfunction affecting the helicopter and the airspeed it could maintain.
[40] Maintenance records indicated the pilot last operated a Christen Eagle II in September 2021 and may have operated a T6 Harvard (fixed wing aircraft) for a total of about 7 hours in the previous 12 months. It could not be determined the extent to which the pilot was the pilot in command during these flights.
[41] ATSB investigation AO-2012-021 identified that a door opening in-flight will not adversely affect control of the helicopter.
[42] The POH stated that each R22 door weighs 5.2 lb (2.35 kg).
[43] RHC safety notice SN-01 Inadvertent actuation of mixture control in flight detailed ‘cases have been reported where a pilot inadvertently pulled the mixture control instead of the carb heat or other control resulting in complete engine stoppage’.
[44] RHC advised that, at maximum continuous power, the throttle is normally open about 75%.
[45] All engines in R22 and R44 helicopters are derated in terms of maximum continuous power, by the pilot following the ‘Limit Manifold Pressure Chart’ in the respective POH. However, the engine is capable of providing more power if required, until the throttle is fully open. This was primarily incorporated to increase helicopter performance at higher altitudes, and to improve reliability and overhaul life. This is further detailed in the online articles Unlocking the mysteries of Robinson’s derated engines and No Ice, Thank You.
[46] The carburettor air temperature and manifold pressures gauges required monitoring by the pilot as there were no warning lamps or horns that would alert the pilot to either being in the range indicative of icing.
[47] In December 2014, RHC published service letter SL-66 Full Throttle Caution Light Kit, which offered an optional field installation kit for a full throttle caution light that illuminates when the engine is approaching full throttle. This would alert the pilot that lowering the collective may be required to avoid low rotor RPM. VH-RAS did not have this kit installed.
[48] The R22 vertical speed indicator has a maximum indication of 2,000 ft/min.
[49] Coning of main rotor blades: the upwards movement of the main rotor blades while they are rotating. This is usually in response to an increase in aerodynamic force as a result of a control input from the pilot. It is more pronounced at high weights and/or low main rotor speed.
[50] R22 main rotor blade skins were changed from stainless steel to aluminium, around 2011, to reduce corrosion and disbonding and improve dent resistance. VH-RAS was fitted with aluminium blades at manufacture.