Executive summary
What happened
On 11 March 2020, a Cessna 404 aircraft, registered VH-OZO, was being operated by Air Connect Australia to conduct a passenger charter flight from Cairns to Lockhart River, Queensland. On board were the pilot and 4 passengers, and the flight was being conducted under the instrument flight rules (IFR).
Consistent with the forecast, there were areas of cloud and rain that significantly reduced visibility at Lockhart River Airport. On descent, the pilot obtained the latest weather information from the airport’s automated weather information system (AWIS) and soon after commenced an area navigation (RNAV) global satellite system (GNSS) instrument approach to runway 30.
The pilot conducted the first approach consistent with the recommended (3°) constant descent profile, and the aircraft kept descending through the minimum descent altitude (MDA) of 730 ft and passed the missed approach point (MAPt). At about 400 ft, the pilot commenced a missed approach.
After conducting the missed approach, the pilot immediately commenced a second RNAV GNSS approach to runway 30.
During this approach, the pilot commenced descent from 3,500 ft about 2.7 NM prior to the intermediate fix (or 12.7 NM prior to the MAPt). The descent was flown at about a normal 3° flight path, although about 1,000 ft below the recommended descent profile. While continuing on this descent profile, the aircraft descended below the MDA. It then kept descending until it collided with terrain 6.4 km (3.5 NM) short of the runway. The pilot and 4 passengers were fatally injured, and the aircraft was destroyed.
What the ATSB found
The weather conditions when the aircraft reached the MAPt for the first approach could not be determined. It is possible that the conditions were better than the landing minima at that point but then deteriorated as the approach continued and when the aircraft was at a lower altitude.
The indicated airspeed during the latter part of the first approach was about 140 kt, which significantly exceeded the operator’s preferred speed after the final approach fix (FAF) (about 110 kt) and the operator’s stabilised approach criteria speed (about 110 kt at 300 ft above aerodrome elevation). Whether the pilot made the decision to conduct the missed approach based on the weather conditions, airspeed, descent rate or some combination of those factors could not be determined.
The aircraft probably entered areas of significantly reduced visibility during the second approach. In particular, there was a period of heavy rainfall at the airport after the first approach, and it is likely the aircraft entered the rain during the second approach.
There was no evidence of any conditions or circumstances likely to induce a medical problem or incapacitation for the pilot and the aircraft appeared to be in controlled flight up until the time of the impact. There was also no evidence of any aircraft system or mechanical anomalies that would have influenced the accident. Therefore, based on the available evidence, the accident was very likely the result of controlled flight into terrain (CFIT).
The most likely scenario to explain the descent 1,000 ft below the recommended descent profile on the second approach could not be determined. Regardless of the exact scenario, it is evident from the continued descent that the pilot did not effectively monitor the aircraft’s altitude and descent rate for an extended period.
In addition, when passing the FAF (5 NM prior to the MAPt), the aircraft significantly exceeded the operator’s required (lateral) navigational tolerance for the instrument approach for an extended period. This should have resulted in a second missed approach but, although the pilot was correcting the lateral deviation, a missed approach was not conducted. The aircraft’s speed after the FAF also increased to 140 kt, before increasing to 150 kt towards the end of the flight.
The ATSB found that the pilot was probably experiencing a very high workload during periods of the second approach. In addition to the normal high workload associated with a single pilot hand flying an approach in instrument meteorological conditions (IMC), the pilot’s workload was elevated due to conducting an immediate entry into the second approach, conducting the approach in a different manner to their normal method, the need to correct lateral tracking deviations throughout the approach, and higher than appropriate speeds in the final approach segment.
The pilot had the required qualifications and had been regularly logging RNAV GNSS approaches, although these approaches were almost all conducted in visual meteorological conditions. However, their workload was potentially further exacerbated by having limited recent experience in conducting RNAV GNSS approaches in IMC.
The aircraft had sufficient fuel to conduct the flight from Cairns to Lockhart River and return, with additional fuel for holding on both sectors if required. In addition, there was no evidence to indicate any organisational or commercial pressures on the pilot to complete the flight, but the extent to which self-imposed pressures or incomplete knowledge of procedural requirements influenced the pilot’s performance could not be reliably determined.
The aircraft was not fitted with a terrain avoidance and warning system (TAWS). Given the aircraft’s descent profile on the second approach, if a TAWS had been fitted and been operational, it would have provided the pilot with both visual and aural alerts of the approaching terrain for an extended period.
There was no requirement in Australia for piston-engine aeroplanes (such as VH-OZO) to be fitted with a TAWS. Although the Civil Aviation Safety Authority (CASA) had been considering changes to TAWS requirements since 2008, the Australian requirements at the time of the accident for some types of small aeroplanes being used for air transport operations were less than those of comparable countries and they were not consistent with International Civil Aviation Organization (ICAO) standards or recommended practices.
More specifically, although there was a TAWS requirement in Australia for turbine-engine aeroplanes carrying 10 or more passengers under the IFR, there was no requirement for piston-engine aeroplanes authorised to carry 10 or more passengers (an ICAO standard adopted as a requirement by many comparable countries) and no requirement for turbine-engine aeroplanes authorised to carry 6 to 9 passengers (an ICAO recommended practice adopted as a requirement by many comparable countries). However, even if these changes had been introduced in Australia prior to the accident, it is unlikely they would have resulted in an aeroplane such as VH-OZO being fitted with a TAWS.
The aircraft was fitted with 2 Garmin GNS 430W GPS units that provided navigation and radio communication capability. As part of the unit’s navigation capability, there was also a terrain awareness function capable of providing visual pop-up terrain alerts. However, that functionality was not to the same standard required for a TAWS installation. It could not be determined whether this function was selected on by the pilot during the accident flight.
Although the GNS 430W unit was suitable for an RNAV GNSS approach and other non-precision instrument approaches, it did not provide vertical guidance information, which would have explicitly indicated that the aircraft was well below the recommended descent profile.
CFIT accidents have been a significant problem over many years, although the rate of such accidents has been decreasing. However, risk factors still remain, particularly for smaller operators. Ideally, in order to minimise the risk of CFIT, operators conducting passenger transport operations under the IFR would use aircraft fitted with a TAWS and/or have a GPS/navigational system that provides vertical guidance during non-precision instrument approaches.
Nevertheless, even without these systems, there are other means available for such operators to minimise CFIT risk. In this case, the operator had specified a flight profile for straight-in instrument approaches and stabilised approach criteria in its operations manual, and encouraged the use of stabilised approaches, but there were limitations with the design of these procedures.
In particular, the operator’s stabilised approach criteria specified an applicable height of 300 ft above aerodrome elevation for operations in IMC. A similar problem has also been identified in multiple other operators conducting passenger transport operations under the IFR. Although an applicable height of 1,000 ft in IMC has been widely recommended by ICAO and many other organisations for over 20 years, CASA had not provided formal guidance information to operators in Australia regarding the content of stabilised approach criteria.
There were also limitations with the operator’s other risk controls for minimising the risk of CFIT, including no procedures or guidance for the use of the terrain awareness function on the aircraft’s GNS 430W units, and limited monitoring of the conduct of line operations.
What has been done as a result
On 2 December 2021, Civil Aviation Safety Regulation (CASR) Part 121 (Australian air transport operations – larger aeroplanes) and CASR Part 135 (Australian air transport operations – smaller aeroplanes) commenced. Associated with these regulations, piston-engine aircraft being used for air transport with a maximum operational passenger seat configuration (MOPSC) of 10 or more were required to have a TAWS and operate under Part 121, with the applicable dates dependent on the MOPSC and other factors.
In December 2021, CASA also published guidance material for CASR Part 121 and Part 135. This included guidance information about stabilised approach criteria, including advice regarding applicable heights for stabilised approach criteria in IMC, including an example height of 1,000 ft above aerodrome elevation in IMC.
Associated with the introduction of CASR Part 135 in December 2021, air transport operators of smaller aeroplanes were required to conduct a flight crew member proficiency check at intervals of 6 months (for IFR or night VFR operations) or 12 months (for day VFR operations).
Safety message
All operators conducting air transport operations under the IFR should evaluate the risk of CFIT in their operations. In addition, any such operators that do not currently have a TAWS fitted to their aircraft should recognise the substantial benefits of a TAWS, and be actively seeking to install a TAWS to maximise the safety of their operations.
In addition, there are many other lessons for operators of small aircraft to reduce their CFIT risk. These include:
- If a TAWS is not currently viable but they have aircraft with a GNS 430 or similar system that provides a terrain awareness function, fully understand the nature and limitations of this function and develop procedures and guidance for pilots about its operation (particularly for instrument approaches or operations in IMC).
- If not already fitted, actively seek to upgrade their GPS/navigational system to one that provides vertical guidance information on non-precision instrument approaches.
- Develop (or review) flight profiles for instrument approaches that provide clear guidance regarding the expected configuration, speed and other requirements at key stages of the approach.
- Develop (or review) stabilised approach criteria in line with best-practice industry guidance and ensure that the applicable heights or reference points are suitable for straight-in approaches and operations in IMC.
- Review the frequency and content of flight crew member proficiency checks to ensure they provide sufficient opportunities to monitor the way instrument approaches are being conducted during line operations (noting that such checks for IFR operations conducted under CASR Part 135 are now required every 6 months). In addition, such operators should consider options for obtaining and reviewing recorded flight data of normal line operations for continuous learning purposes.
The occurrence
Overview
On 11 March 2020, a Cessna 404 aircraft, registered VH-OZO, was being operated by Air Connect Australia to conduct a passenger charter flight from Cairns to Lockhart River, Queensland. On board were the pilot and 4 passengers. The flight was being conducted under the instrument flight rules (IFR).[1]
Consistent with the forecast, there were areas of cloud and rain that significantly reduced visibility at Lockhart River Airport. After arriving at Lockhart River, the pilot commenced an area navigation (RNAV) global satellite system (GNSS) instrument approach to runway 30. The aircraft descended to an altitude of about 400 ft before the pilot conducted a missed approach. The pilot immediately commenced a second RNAV GNSS approach to runway 30, and during the descent the aircraft collided with terrain.
Planned flight
The passengers were contracted to carry out work at the local school at Lockhart River. The client arranged with the operator for the aircraft to depart Cairns at 0730 Eastern Standard Time[2] on 11 March 2020, wait on the ground at Lockhart River for about 5 hours, then depart at 1430 with the same passengers for the return flight. The operator assigned the pilot who regularly conducted the operator’s charter flights.
For the arrival at Lockhart River, the forecast weather was for light winds and rain and low cloud with periods of visibility reducing to 3 km in rain. There was also a 30% probability of thunderstorms. The pilot had submitted a flight notification, which specified IFR and capability for an RNAV instrument approach. The aircraft had sufficient fuel to conduct an approach at Lockhart River and return to Cairns and hold at Cairns for 1 hour if required.
Flight to Lockhart River
The aircraft departed Cairns at 0719 and tracked for the first planned waypoint on climb to its cruise level of 10,000 ft above mean sea level. Based on the forecast winds, the estimated time of arrival at Lockhart River was 0852. As the flight progressed, the pilot amended the estimated time of arrival to 0904. The aircraft’s track during the flight is shown in Figure 1.
Figure 1: VH-OZO recorded flight path from Cairns to Lockhart River, Queensland
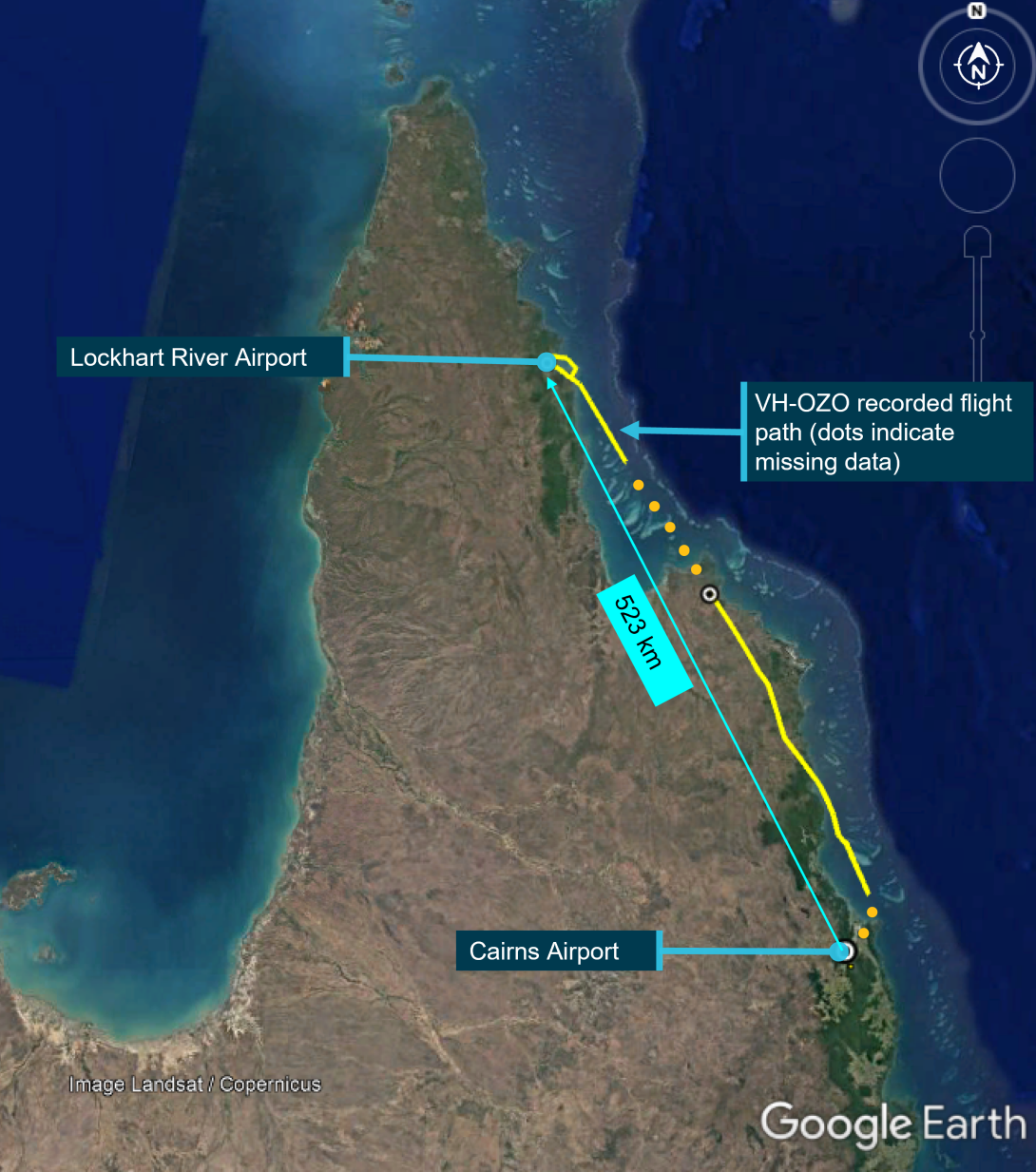
Source: Google Earth overlaid with OzRunways data, annotated by the ATSB
At 0836, the pilot advised air traffic control that the aircraft was approaching top of descent, then tracking direct for the runway 30 RNAV GNSS instrument approach at Lockhart River, and the pilot requested traffic information. The controller responded there was no reported IFR traffic. At 0840, the pilot reported leaving 10,000 ft on descent and, at 0842, the controller advised the pilot of the very high frequency (VHF) and high frequency (HF) radio frequencies applicable to the rest of the flight. That was the controller’s last contact with the pilot and no further routine interactions with the controller were expected.[3]
During descent, the pilot transmitted on the common traffic advisory frequency (CTAF) for Lockhart River to activate the runway lighting for a period of 30 minutes. At 0852, the aerodrome frequency response unit (AFRU) broadcast ‘Lockhart River CTAF, runway lights are on’.
At about this time, the pilot very likely obtained weather information from the automated weather information service (AWIS) via VHF radio. Notes taken by the pilot indicated the wind was calm, visibility was at least 10 km, there was broken cloud[4] at 1,800 ft, broken cloud at 3,500 ft and overcast cloud at 5,300 ft, and the QNH was 1,008 hPa (see Automated weather information service).
At about 0857, the aircraft levelled off at 5,500 ft. At this time the aircraft was heading to waypoint LHREB, one of 3 initial approach fixes (IAFs) for the RNAV GNSS instrument approach to runway 30 (Figure 2). The weather information indicated that the conditions were better than the landing minima (which were a cloud ceiling of 730 ft and visibility 4,200 m).[5]
Figure 2: Lockhart River RNAV GNSS runway 30 approach chart
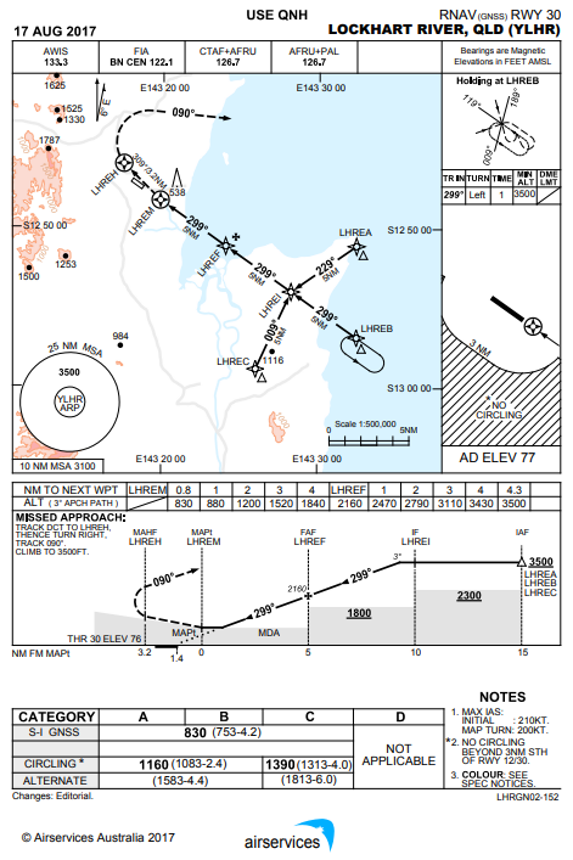
Source: Airservices Australia, annotated by the ATSB
First approach at Lockhart River
Figure 3 depicts the aircraft’s recorded flight track for the first approach and missed approach at Lockhart River. The altitudes described throughout the report are truncated to the nearest 100 ft. [6]
At 0859:38, the aircraft passed abeam LHREB, commenced descent from 5,400 ft and turned left to track to the runway in accordance with the RNAV GNSS procedure. At 0901:25, the pilot made a radio broadcast on the CTAF, advising the aircraft was 10 NM[7] to the south-east of the aerodrome, inbound to runway 30 and on descent passing 4,000 ft. Shortly afterwards, the aircraft passed the intermediate fix (IF) LHREI at 4,000 ft.
Figure 3: Flight track of VH-OZO during first RNAV GNSS approach at Lockhart River Airport with times, feature labels, and approach parameters superimposed
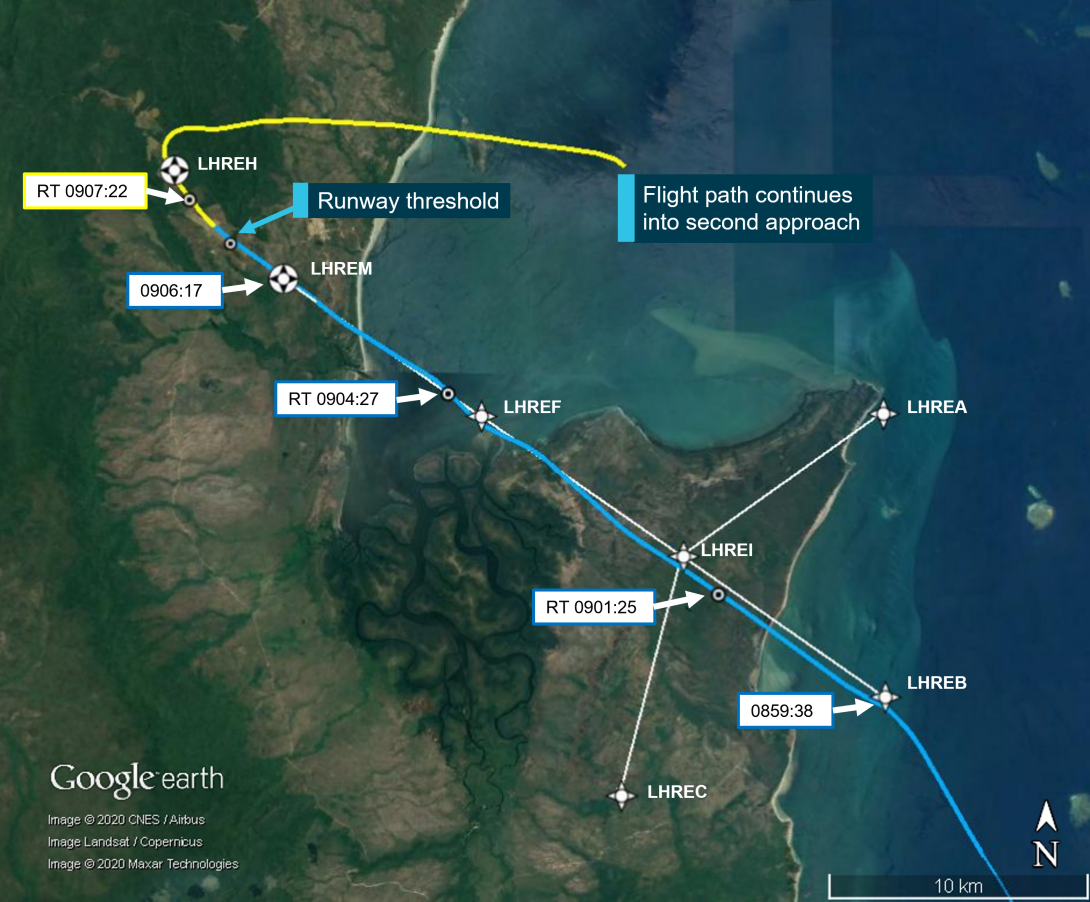
Source: Google Earth overlaid with OzRunways data, annotated by the ATSB
At about 0903, one of the passengers sent a text message that contained an image of conditions outside the aircraft (Figure 4). At that time, the aircraft was over halfway between LHREI and the final approach fix (FAF) LHREF, at an altitude between 3,100 and 2,500 ft. The photograph had been taken through a passenger window on the right side of the aircraft cabin and, although there was significant cloud in the vicinity, some terrain/coastline was visible near the intersection of the wing’s leading edge and the engine cowl.
Figure 4: Image recorded by a passenger looking forward over the right engine and sent via text message at 0903
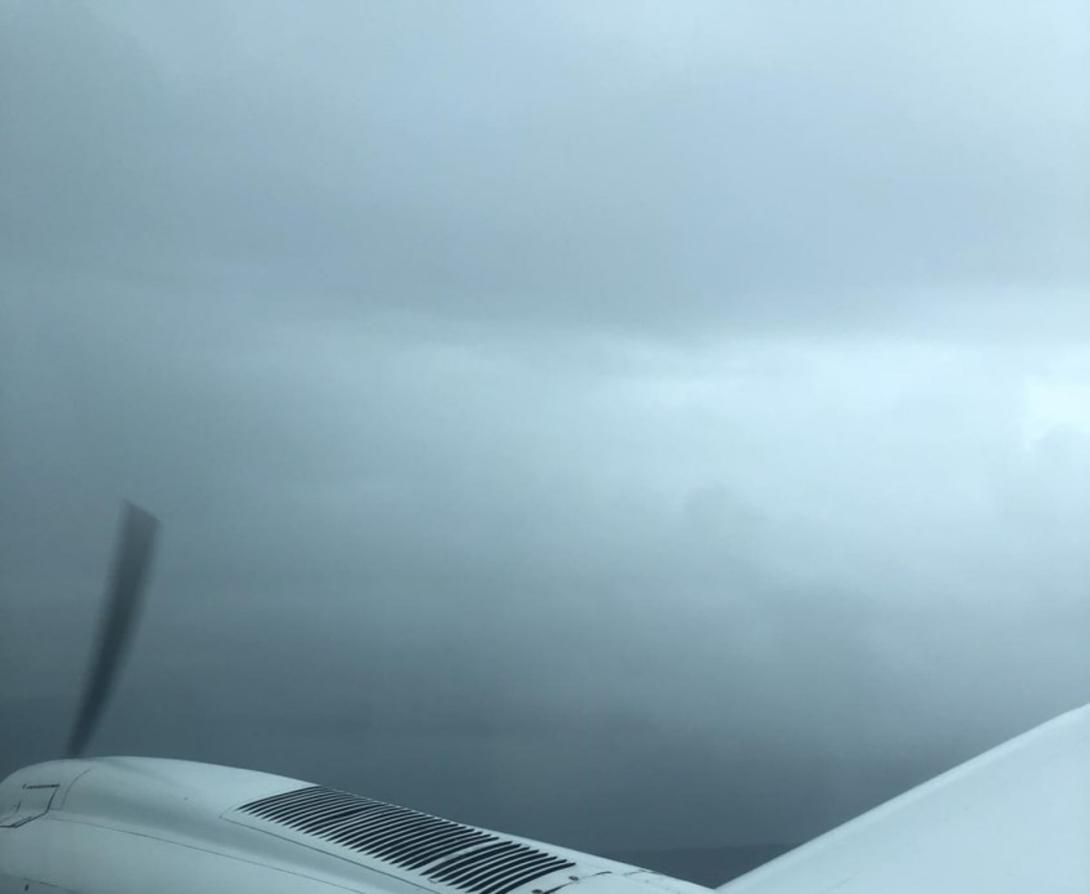
Source: Supplied, lower section of image cropped by the ATSB
The aircraft continued the descent on the approach track and passed LHREF on descent through 2,300 ft. At 0904:27, the pilot broadcast on the CTAF that the aircraft was at 5 NM and on final (approach) to runway 30.
The minimum descent altitude (MDA) was 730 ft.[8] The aircraft arrived at the missed approach point (MAPt) LHREM at 0906:17 on descent through about 600 ft. The descent continued to about 400 ft then, about 1,000 m from the runway, the aircraft started to climb. The aircraft was passing 600 ft as it crossed the runway threshold in the early stages of a missed approach. In accordance with the missed approach procedure, the aircraft was turned slightly right to track towards the turning fly-over waypoint LHREH.
At 0907:22, the pilot broadcast on the CTAF that they were conducting a missed approach for runway 30, tracking to the west then turning back to the east and climbing towards 3,500 ft (as specified for the missed approach procedure). After passing LHREH at 0907:43 on climb through 1,200 ft, the aircraft turned right to track east as prescribed by the approach chart.
At 0909, the pilot contacted Flightwatch[9] on HF and advised:
[VH-OZO] conducting a missed approach runway three zero [30] at Lockhart River, and we’ll be joining the approach on runway three zero [30], ops normal time two three three zero [2330]
The middle part of this radio transmission, as recorded by Airservices Australia, was unclear, which is not uncommon for HF radio communication.
Second approach at Lockhart River
The aircraft continued the climb to 3,800 ft before descending to level out at 3,500 ft, heading towards the closest IAF, LHREA. At 0912:51, the AFRU recorded runway lights on, consistent with the pilot reactivating the runway lights for another 30-minute period. About 2.0 NM prior to reaching LHREA, at 0913:53, the aircraft commenced a right turn towards the IF, and initially was right of the inbound track to LHREI (Figure 5).
Figure 5: Flight track of VH-OZO during second RNAV GNSS approach at Lockhart River Airport with times, feature labels, and approach parameters superimposed
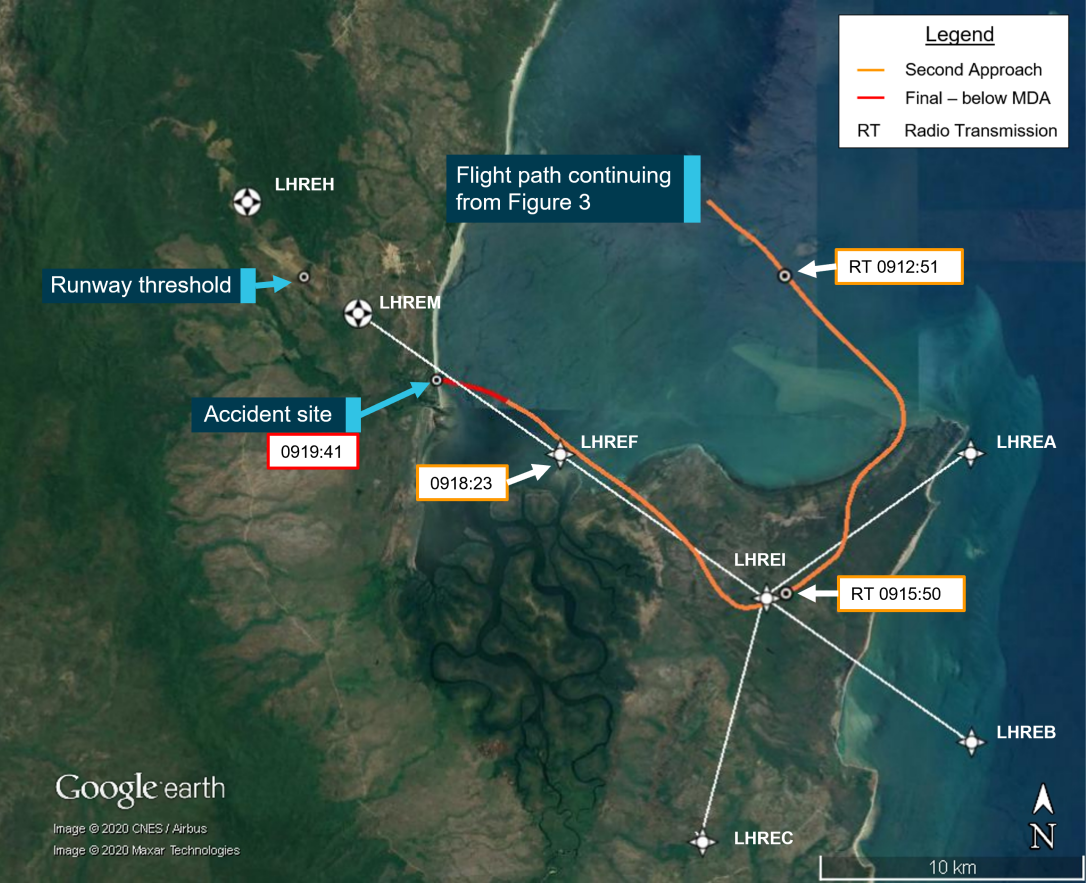
Source: Google Earth overlaid with OzRunways data, annotated by the ATSB
At about 0914, while the aircraft was tracking towards LHREI on a south-westerly heading at 3,500 ft, an image was uploaded to social media by one of the passengers (Figure 6). The camera was oriented to the west, which was in the general direction of Lockhart River. An associated message indicated very low visibility and that the pilot was circling while waiting for a break in the weather. Another passenger sent a text message at 0914 stating that the first attempt at landing was unsuccessful, the runway was not visible and there was heavy rain.
Figure 6: Image recorded by a passenger looking over the right wing and uploaded to social media at 0914
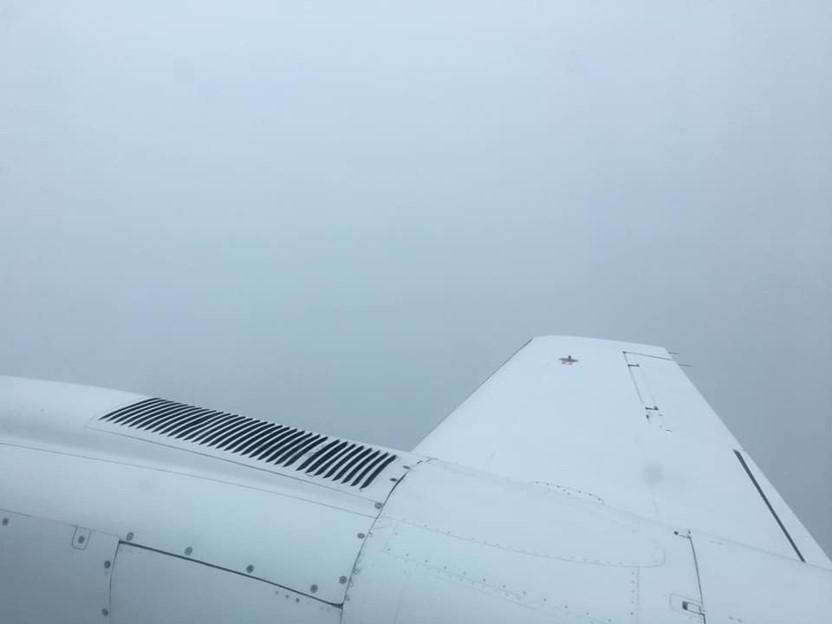
Source: Supplied
At about 0914:43, when about 2.7 NM from LHREI, the aircraft started descending from 3,500 ft. At this time, the aircraft was tracking towards the initial approach track between LHREA and LHREI (Figure 5).
At 0915:50, the pilot made another inbound broadcast on the CTAF advising:
ten miles [10 NM] to the south-east on descent passing three thousand eight hundred [3,800 ft] correction two thousand eight hundred [2,800 ft], straight-in approach runway three zero [30], circuit area two one [time 0921].
The recorded height was about 2,800 ft at this time.
The aircraft continued descending and passed over LHREI and turned right to fly parallel to the intermediate approach track at about 2,800 ft. According to the recommended flight profile for a 3° approach (Figure 2), the aircraft should have descended from 3,500 ft at about 4.2 NM from the FAF (9.2 NM from the MAPt). At this point, the aircraft was at about 2,500 ft.
The descent continued at a similar gradient to the first approach although at about 1,000 ft lower than that approach. About halfway between LHREI and LHREF, the aircraft descended below the intermediate segment minimum safe altitude of 1,800 ft and continued to descend on the same descent profile.
When the aircraft passed LHREF at 0918:23, it was on descent through about 1,100 ft (below the 3° approach profile height of 2,160 ft). The aircraft was right of the final approach track and, shortly after passing LHREF, the aircraft started turning back towards the final approach track.
From LHREF to LHREM, the altitude limitation was the MDA of 730 ft and, at 09:18:55, the aircraft was approaching 700 ft. The aircraft then descended below the MDA and, soon after, the aircraft’s flight path crossed the final approach track (on a ground track about 20° left of the final approach track).
Collision with terrain
The aircraft’s track and descent continued until it impacted a sand dune on the coastline at about 0919:41. The pilot and 4 passengers were fatally injured, and the aircraft was destroyed. Due to the impact forces, the accident was not survivable.
Context
Pilot information
Qualifications and experience
The pilot held a commercial pilot licence (aeroplane) with an instrument rating and multi-engine aeroplane endorsement. They had recorded a total of 3,220 hours before the accident flight.
The pilot obtained the multi-engine endorsement in June 2014 (on a Cessna 310 aircraft), and had accrued 1,177 hours on multi-engine aircraft, including 399 hours on the Cessna 404 aircraft type. In June 2014, the pilot also obtained their initial (multi-engine) instrument rating, and their total instrument time was recorded as 148 hours.
The pilot operated as a commercial pilot in remote locations for about 5 years. Up until March 2016, they operated single-engine aircraft. In March 2016 they received training and were found competent on the Piper PA31 aeroplane type. Between March 2016 and February 2018, the pilot conducted flights for a charter company that operated Cessna 310 and Piper PA-31 aircraft, usually under visual flight rules (VFR[10]) with occasional instrument flights. They were approved by the Civil Aviation Safety Authority (CASA) as chief pilot of this operator in December 2016.
From October 2018, the pilot was employed by Air Connect Australia on a casual basis. Prior to joining the operator, the pilot’s recorded total flying time was 2,800 hours. The pilot completed induction then conducted a flight for type-specific training in a Cessna 421 from an independent CASA-approved flight examiner that the operator frequently used for proficiency checks. This flight was about 1.6 hours and the examiner recalled that the pilot managed the transition to the 400-series Cessna without any problems. Other than the pressurisation system in the Cessna 421, the examiner considered it was operationally equivalent to the unpressurised Cessna 404.[11]
Following this type-specific training, the chief pilot of Air Connect Australia supervised the pilot on 4 flight sectors in VH-OZO and conducted an operator proficiency check (OPC) over 2 further sectors on 29 October 2018. The chief pilot noted that the pilot’s planning was satisfactory, and operation of the aircraft was above standard.
From November 2018 to the accident flight, the pilot was based in Cairns and conducted most of the operator’s charter flights, normally in VH-OZO. In December 2019, the chief pilot organised for the pilot to undertake some supervised flying at night with an instructor in a Piper PA-44 Seminole in order for the pilot to maintain night recency. The operator rarely conducted night flights.
In the 90 days prior to the accident (11 March 2020), the pilot had conducted 59 flights (60 flight hours), all in VH-OZO. This included 4.5 hours recorded instrument flying time. In the last 30 days, the pilot had conducted 12 flights (13.5 flight hours), including 1.0 hour recorded instrument flying time. The most recent flights were on 18 February 2020.
Since joining the operator in late 2018, the pilot had logged 69 RNAV GNSS approaches to various aerodromes. These included 21 RNAV GNSS approaches in the previous 6 months, 12 in the previous 90 days, and 2 in the previous 30 days (with the last on 18 February 2020). Only one of the approaches in the previous 6 months was conducted to some extent in instrument meteorological conditions (IMC),[12] and this approach resulted in a missed approach (see Prior missed approach during an RNAV GNSS approach (22 January 2020).
Lockhart River experience
Since the start of 2019, the pilot had flown into Lockhart River 8 times, 6 of which were logged as RNAV GNSS approaches, with the most recent being on 14 October 2019.
The recorded data for previous RNAV GNSS approaches into Lockhart River were reviewed by the ATSB, with the details provided in Table 1. A review of recorded weather information indicated that none of these previous approaches at Lockhart River were conducted in IMC. For the 17 January 2019 approach, there may have been reduced visibility in the early part of the approach.[13]
Table 1: Pilot’s prior flights to Lockhart River 2019–2020
Date | Departure location | Approach type recorded | ATSB comments |
17 January 2019 | Cairns | RNAV GNSS | Aircraft passed over MAPt for runway 30 at 1,500 ft and then conducted a circling approach to land on runway 12 |
21 March 2019 | Cairns | RNAV GNSS | Missing flight data for the approach and landing though appeared to be heading for runway 30 IAF |
23 March 2019 | Cairns | RNAV GNSS | Flight data shows an RNAV approach to runway 30 from the FAF but missing flight data before that point |
24 March 2019 | Coen | RNAV GNSS | Flight data shows an RNAV approach to runway 30 from the FAF but missing flight data before that point |
24 March 2019 | Coen | VFR | Logged as VFR flight, so data was not reviewed |
18 April 2019 | Cairns | VFR | Logged as VFR flight, so data was not reviewed |
9 October 2019 | Cairns | RNAV GNSS | No flight data available |
14 October 2019 | Cairns | RNAV GNSS | No flight data available |
Proficiency checks and flight reviews
The pilot conducted initial instrument flight training in 2014. During training it was noted that the pilot needed to scan faster, improve situation awareness and improve radio phraseology. On one simulator training exercise it was noted the pilot was too high on the RNAV GNSS MDA and minimum altitudes.
The pilot did not pass their first attempt at attaining an instrument flight rating (in a Cessna 310) on 2 June 2014 for not maintaining altitude within +100 ft and -0 ft at the MDA, not using accepted navigation procedures, not being within half-scale deflection of glideslope, and not demonstrating sound command judgement. On the next day, 3 June 2014, the pilot passed on their second attempt.
Under Civil Aviation Safety Regulation (CASR) 61.650, pilots need to have completed an instrument proficiency check (IPC) in the previous 12 months to fly a multi-engine aircraft under the instrument flight rules (IFR). The IPC must also be done in a multi-engine aircraft of the same category.
The pilot undertook 6 IPCs with 3 different independent CASA-approved flight examiners between 2016 and 2019. These are detailed in Table 2.
Table 2: Pilot instrument proficiency checks 2016–2019
Date | Outcome |
17 March 2016 | Competent |
14 May 2017 | Not competent on a ground component (underpinning knowledge) with no assessment of flying capability |
27 July 2017 | Competent |
4 August 2018 | Not competent due to misread of altimeter by 1,000 ft (under-read) |
5 August 2018 | Competent |
7 August 2019 | Competent |
The pilot’s last 3 IPCs were conducted on 4 August 2018, 5 August 2018 and 7 August 2019, all with the same CASA-approved flight examiner (who also conducted the IPC in March 2016).
On 4 August 2018, the pilot did not pass the IPC due to misreading the altimeter. The flight examiner recalled that they were on descent to the minima on a circling approach and, when the aircraft was at 1,000 ft above the MDA, the pilot asked whether they were visual. It then became apparent that the pilot had misread the altimeter by 1,000 ft (that is, they thought the aircraft was 1,000 ft lower than it was). The pilot successfully passed the check the following day.
The pilot’s most recent IPC was conducted on 7 August 2019 and was valid until 7 August 2020. The flight examiner who conducted this check was selected by the pilot; it was not the flight examiner regularly used by the operator (and who was familiar with the Air Connect Australia operations manual and could also conduct OPCs).
For each of the IPCs in 2018 and 2019, the pilot conducted a training flight with an instructor prior to the test flight, using the same Cessna 310 aircraft as in the test flights. The instructor commented that the pilot flew significantly better in 2019. Between the 2 checks in 2018 and 2019, the Cessna 310 aircraft was fitted with a GPS/navigational system and electronic flight instruments comprising an attitude display indicator (ADI) and a horizontal situation indicator (HSI). The ADI displayed aircraft attitude information, together with a secondary display of air data information (airspeed, altitude and vertical speed). The ADI and HSI each provided course and advisory vertical guidance during RNAV GNSS approaches.[14]
As already noted, the pilot undertook an operator proficiency check (OPC) on 29 October 2018 before commencing line operations with Air Connect Australia. This was the last OPC carried out on the pilot (see also Operator proficiency checks).
Between February and June 2018, prior to joining the operator, the pilot undertook training with an airline in a multi-crew environment and high-performing (turboprop) aircraft. Although the pilot obtained high marks in theory and written tests, they did not obtain satisfactory ratings during 3 proficiency assessments in a simulator (with remedial training given after each of the first 2 assessments). A common identified problem was instrument approaches, with issues identified including inefficient instrument scan, fixation (on some parameters), speed control, workload management, insufficient situational awareness and ineffective profile management. The ATSB notes that the training and checking environment at the airline was different to the pilot’s previous experience and the operational environment at Air Connect Australia.
Observations of the pilot’s approach to safety
The chief pilot (and managing director) of Air Connect Australia described the pilot as being a good pilot who would not have gone into an approach if they thought the weather was going to be poor, and that there was never any pressure to fly in poor weather. The pilot was trusted to make safety decisions, which would be supported by the chief pilot. This was consistent with the recollection of a previous pilot who flew with the operator, who reported that there was never any operational pressure (from the operator’s key personnel).
Another pilot stated that the pilot of the accident flight had ‘good stick and rudder skills’ and that everything was done ‘by the book’. It was also reported that the pilot had not expressed any concerns about the operator, including its approach to safety.
Former colleagues from when the pilot was chief pilot at a previous operator described the pilot as smart, diligent, and methodical with good knowledge of the rules and regulations. They reported that the pilot did not take shortcuts or unnecessary risks and had good hand-flying skills.
With reference to instrument approaches, one pilot advised that they had many conversations with the pilot of the accident flight regarding aircraft accident reports and safety, and the pilot of the accident flight had stated that they would conduct instrument approaches using the published constant-descent profile and would not intentionally deviate below published segment minimum safe altitudes in order to get visual early in an approach.
During January 2020, the pilot spent a week conducting a series of charter flights between Aurukun and Weipa, Queensland, in VH-OZO with the same group of passengers (see also Prior missed approach during an RNAV GNSS approach (22 January 2020)). Their perception was that the pilot was a good, competent pilot who was diligent, professional, and responsible and that they never felt unsafe. They also advised that they observed the pilot reviewing forecast and actual weather conditions regularly and that the pilot would delay flights due to weather conditions if necessary. Some of the passengers reported observing the pilot make weather-based decisions and did not display any indications of external pressure to fly in poor weather. One of the passengers reported that the pilot had said they would only ever make 2 attempts at landing and, after that, would return to the departure aerodrome or divert to an alternate.
Recent history
The pilot had recently returned from annual leave, with their last flights before leave conducted on 18 February 2020. The 3 days prior to the accident flight were reported to be uneventful. It was described that the pilot ate and exercised regularly and had been sleeping well with a standard time to sleep about 2230 and wake time about 0700–0730. The night before the accident flight, the pilot went to bed at about 2230 and woke at about 0530. There was nothing of note from the pilot’s recent history to suggest they were experiencing a level of fatigue known to affect performance.
The pilot was notified about the 11 March 2020 flight a few days in advance. It was reported that the pilot had been in a good mood in the days prior to the flight and was looking forward to flying again. On the day before the flight, the pilot went to Cairns Airport and started the aircraft’s engines to re-familiarise themselves and ensure everything was ready for the next day’s flight.
Medical information
The pilot’s Class 1 Aviation Medical Certificate was renewed on 14 February 2020 and was valid until 14 February 2021. There were no indications of any significant medical problems in the pilot’s aviation medical records. There was no evidence to suggest the pilot had any current or ongoing medical issues at the time of the accident.
A post-mortem examination was conducted by a forensic pathologist on behalf of the Queensland Coroner. The pathologist found that there was ‘No obvious natural disease to contribute to the cause of death within limits of examination …’. Forensic toxicology screening returned negative results (that is, no alcohol or substances were detected). The sample was unsuitable for analysis for carbon monoxide.
Aircraft information
General information
The Cessna 404 Titan is an unpressurised, low-wing, twin piston-engine aircraft with retractable landing gear. The maximum take-off weight (MTOW) is 3,810 kg, and the aircraft was certified to be flown by a single pilot.
VH-OZO was manufactured by the Cessna Aircraft Company in 1980. It was reported that the aircraft was first operated in Australia before being transferred to Papua New Guinea and registered as P2-ALG. In December 2009, a CASA Certificate of Airworthiness was issued, and the aircraft was registered as VH-OZO. At that time, the aircraft’s total time in service was 28,193 hours.
Seating
The type certificate data sheet for the Cessna 404 stated the aircraft type had 11 total seats (2 pilot seats and 9 passenger seats). In 1980, VH-OZO was configured with a modified seating configuration with 13 seats (2 pilot seats and 11 passenger seats).[15]
During an audit of Air Connect Australia in June 2017, CASA identified that the Airplane Flight Manual stated a maximum of 9 passenger seats aft of the pilot seats but there was 11 on the aircraft. In its initial audit response, the operator stated that the seating change was approved many years ago and it was attempting to find supporting documentation. In a subsequent response, the operator stated that it had previously operated and would continue to operate with a maximum of 9 passengers. It noted that the extra seating would remain in the aircraft as it formed part of the aircraft’s current weight and balance data.
During the investigation, the chief pilot confirmed that the operator never operated the aircraft with more than 9 passengers and normally operated with significantly less than 9 passengers.
Photos taken during the accident flight indicated that no passengers were seated in the front right seat next to the pilot.
Aircraft instruments and systems
On arrival into Australia, the aircraft was fitted with aerial geophysical survey equipment and was operated in that configuration until the equipment was removed in March 2012. Concurrently, the aircraft were modified in accordance with an engineering order to install new types of avionics and integrate those with existing units. The post-modification avionics, including existing equipment, consisted of:
- Garmin GMA 340 audio panel
- dual Garmin GNS 430W GPS/Nav/Com units
- dual Garmin GI-106A GPS/VOR/LOC course deviation indicators (CDIs)
- Garmin GTX327 transponder
- Bendix/King KR87 automatic direction finder (ADF) and Bendix/King KI-227 ADF indicator
- Collins HF radio
- Cessna 400B Navomatic autopilot
- Bendix weather radar (monochrome display).
These units were still installed at the time of the accident except for the transponder, which had been replaced by an automatic dependent surveillance-broadcast (ADS-B) compliant unit in April 2017.
VH-OZO was not fitted with a terrain avoidance and warning system (TAWS), nor was it required to be under legislation in place in Australia at the time of the accident. Further information is provided in Terrain avoidance and warning systems.
An assigned altitude indicator was fitted to the aircraft, which was designed to be used as a reminder of the designated altitude. Altitudes could be manually set by means of individual thumb wheels and no aural or visual alerts were provided when reaching or leaving the set altitude. The aircraft did not have an altitude alerting system, nor was it required for the type of aircraft and operation.[16]
The 400B autopilot was one of the standard equipment options for the Cessna 404 type. It could provide pitch and roll control with heading and altitude hold (on command). A navigation function provided the autopilot with inputs from an associated CDI (the GI-106A), which in this case received data from the number‑1 GNS 430W.
For an RNAV GNSS approach, a pilot could ‘couple’ the autopilot for lateral navigation and manage vertical navigation by adjusting the autopilot pitch wheel to achieve the intended rate of descent. For a level segment, the pilot could select altitude hold on reaching the intended altitude. Several pilots who had flown VH-OZO advised that they routinely hand flew instrument approaches due to autopilot constraints.
The aircraft was fitted with the instrumentation required for operations under the IFR. These flight instruments were conventional analogue indicators and reflected the original specifications for the aircraft. The second artificial horizon/attitude indicator and altimeter were located on the right side of the co-pilot panel (far side of the instrument panel relative to the pilot) (Figure 7).
Figure 7: VH-OZO instrument panel
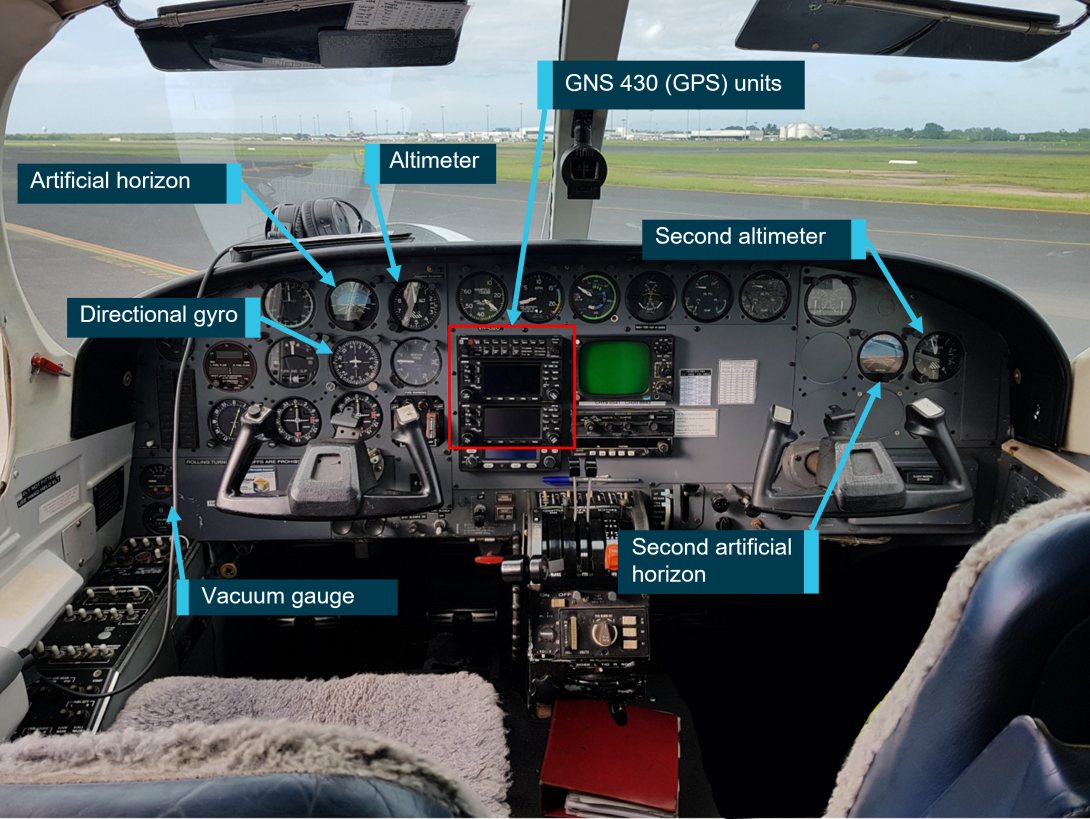
Source: Supplied, annotated by the ATSB
A closer view of the instrument panel is depicted in Figure 8. It had the standard 6 flight instruments directly in front of the pilot’s seat on the left. These include the attitude indicator, which depicts the aircraft’s basic roll and pitch attitude, and the primary performance instruments – altimeter, airspeed indicator and vertical speed indicator (VSI). Below those were 2 (GI-106A CDI) instruments[17] that provided course deviation indication provided either by the GNS 430’s digitally-tuned VOR/localiser and glideslope receiver or GPS input to conduct an RNAV GNSS approach. One CDI instrument was coupled to the aircraft’s number-1 GNS 430W GPS unit and the other to the number-2 GPS unit.
The basis of cockpit design is to have the primary instruments within a small arc of the pilot’s forward line of sight. Navigation systems such as the GPS units may be located next to the primary instruments, as was the case in VH-OZO. While conducting an RNAV GNSS approach, it is imperative that the pilot includes the GPS units in the scan.
Figure 8: Instrument panel of VH-OZO
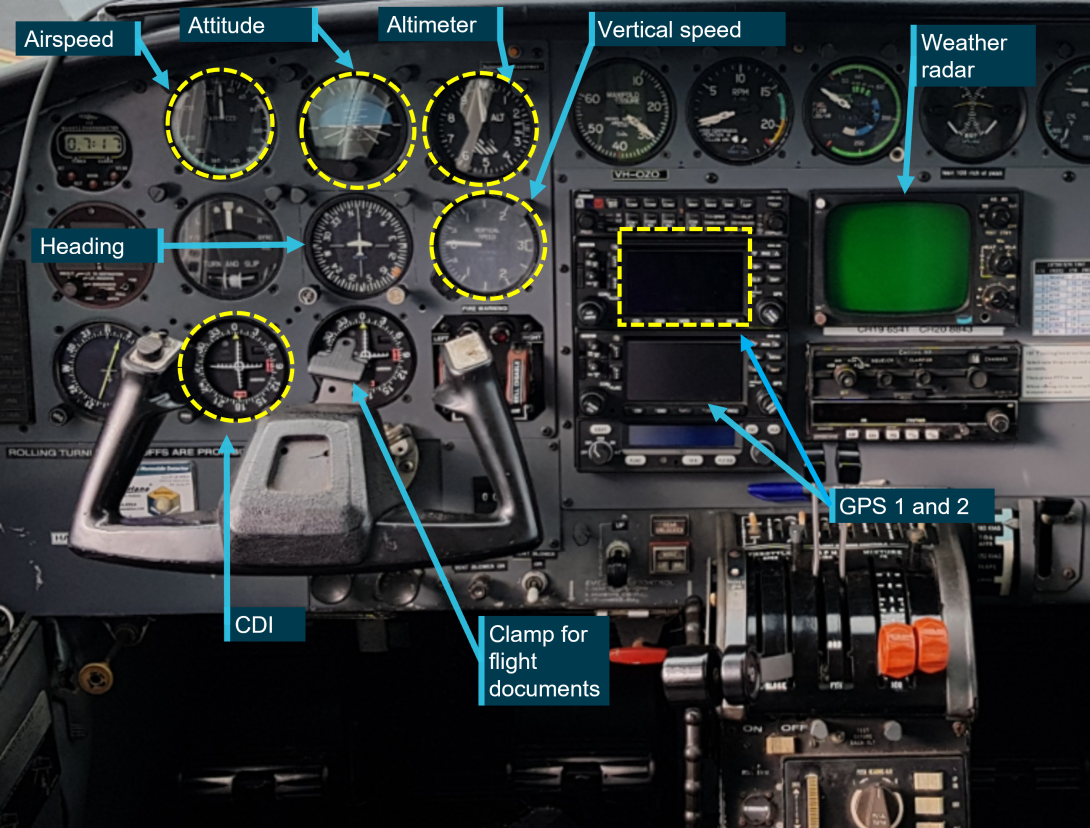
Source: Supplied, annotated by the ATSB
Altimeters
VH-OZO was equipped with two 3-pointer altimeters (Figure 9), including one directly in front of the pilot. They had a 100-ft pointer (long and narrow), 1,000-ft pointer (short and wide) and 10,000-ft pointer (long and thin with a triangle at the end). The diagonal hashing indicated when below 10,000 ft and was gradually covered above that height.
These types of 3-pointer altimeters are very common in general aviation aircraft, including small aeroplanes used for passenger transport activities. Research has shown that such altimeters can be associated with misreading errors, including misreading the altitude by 1,000 ft, although accidents known to be associated with such errors seem relatively rare. Accordingly, such altimeters (and some other altimeter designs) are no longer allowed to be used on air transport certificated aircraft. Further information about requirements and guidance regarding altimeters is provided in Appendix A – Research and guidance regarding design of altimeters.
The aircraft was not fitted with a radio altimeter, nor was it required for the type of aircraft and operation.
Figure 9: Example of the 3-pointer type of altimeter fitted in VH-OZO
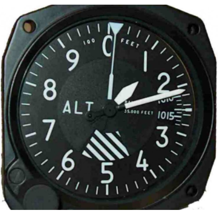
This altimeter shows an altitude of 1,210 ft (10,000 ft pointer indicating 0, 1,000 ft pointer indication 1,000 ft and 100 ft pointer indicating 210 ft.
Source: avioelectronica.com
GNS 430W overview
The Garmin GNS 430W is a panel-mounted unit that provides GPS navigation, instrument landing system or VHF omnidirectional radio range navigation, and VHF radio communication. It was approved for IFR operations, including RNAV GNSS approaches, and was used in conjunction with a CDI.
Although the ‘W’ designated wide area augmentation system capabilities[18] that facilitated GPS approaches with vertical guidance, Australia did not have the associated satellite-based augmentation system to enable this functionality at the time of the accident. As such, the GNS 430W was approved to provide distance and track information only for RNAV GNSS non-precision approaches.
Information was displayed to the pilot on an 8.4 cm by 4.6 cm (240 by 128 pixel) high-contrast colour LCD. A pilot could select the pages and menus to display relevant information during various flight stages. Those included the default navigation page and additional pages including:
- a 2-dimensional representation of terrain relative to the aircraft position
- information for vertical navigation of the aircraft
- a moving map display
- information about the status of the GPS satellite constellation
- information relevant for the navigation and communication functions of the unit.
When used for an RNAV GNSS approach, the navigation page would display a graphic CDI together with the active leg of the approach and 6 user-selectable data fields.[19] After passing the waypoint it was tracking to, the unit would automatically sequence to the next waypoint.
When approaching a waypoint such as an initial approach fix (IAF), if a turn was required, the unit would display the recommended flight path (turn) to intercept the next track segment. The unit would also display a flashing message about 10 seconds prior to the start of the recommended turn, alerting the pilot that a turn was required and the track to intercept.
To use the unit for RNAV GNSS approaches, it was a requirement that the NavData card[20] was valid and the approach procedure was loaded from the database. The operator subscribed to the Jeppesen NavData service that provided monthly updates. It was reported that the pilot updated the NavData card using a laptop computer in the 24 hours prior to the flight. There were no changes in the update that would have been relevant to the accident flight.
The terrain, obstacle and airport terrain database was loaded on a terrain data card. The operator did not subscribe to an update service for terrain/obstacle data. Obstacle data was updated on a 56-day cycle and updates to the terrain database were released on an ‘as-needed’ basis. There was no requirement to have current obstacle or terrain databases to use the GNS 430W for flight under the IFR and/or during an RNAV GNSS approach. A June 2018 photograph of VH‑OZO’s GNS 430W receivers indicated the obstacle database installed at that time was dated October 2011.[21]
Fault detection and exclusion was incorporated into the GNS 430W software to detect satellite failure and exclude failed satellites from usage.
In addition to their experience with VH-OZO, the pilot of the accident flight had experience using GNS 430 units during their time flying Cessna 310 and PA-31 aircraft with a previous operator, which included units with a terrain awareness function.
Garmin TERRAIN function
Garmin TERRAIN was a non-certified[22] terrain awareness system, provided as a standard feature of 400W-series units, to increase pilot situation awareness and help reduce the risk of controlled flight into terrain (CFIT). The functions required a valid 3D GPS position and a valid terrain and obstacle database. Terrain and obstacle information was advisory only and was not equivalent to warnings provided by TAWS. The Garmin 400W Series Pilot’s Guide & Reference manual stated:
CAUTION: The Terrain feature is for supplemental awareness only. The pilot/crew is responsible for all terrain and obstacle avoidance using information not provided by the 400W-series Terrain feature.
When the GNS 430’s terrain page was selected, it presented a 2-dimensional colour-coded display of terrain tiles and obstacles from its database, relative to the aircraft’s current position/altitude. Red (warning) indicated terrain/obstacles above and up to 100 ft below the aircraft’s current altitude, yellow (caution) between 100 ft and 1,000 ft below the current altitude, and black more than 1,000 ft below the current altitude. The terrain page would not normally be selected when conducting an RNAV GNSS approach.
Terrain advisory and alert messages were provided when flight conditions met specific parameters. The advisories/alerts comprised a visual annunciation in the lower left corner of the unit’s LCD display, accompanied by a larger pop-up advisory/alert on the current display page (except the page displaying terrain). To clear the pop-up advisory/alert, the pilot would either acknowledge the message to return to the selected page or acknowledge the advisory/alert and display the terrain page. The system did not provide auditory alerts.
A pilot could use an ’inhibit mode’ to deactivate the terrain advisory/alert message system and pop-up messages would not be generated. The terrain page was still selectable and would display colour-coded terrain and obstacle information relative to the aircraft’s position. Once inhibited, the terrain annunciator field displayed a ‘TER INHIB’ annunciation and the terrain alert system remained deactivated until reselected. The GNS 400W-Series manual stated that the terrain inhibit mode could be used when the advisories/alerts were deemed unnecessary by the pilot. The manual also stated:
Flying VFR into an area where unique terrain exists could cause the system to annunciate a nuisance alert. Pilots should use discretion when inhibiting the TERRAIN system and always remember to enable the system when appropriate.
According to the GNS 400W-Series manual, the terrain system issued a premature descent alert (PDA) when the aircraft was significantly below the normal approach path to a runway. The manual indicated that this alert would activate depending on the aircraft’s height above terrain and distance from the runway threshold (for example, it would be triggered if the aircraft was about 400 ft above terrain when 10 NM from the runway threshold, 350 ft at 5 NM, 320 ft at 4 NM, and 280 ft at 3 NM). PDA alerts were not provided when the aircraft was within 0.5 NM of the runway or less than 125 ft above terrain within 1.0 NM of the runway.
Based on this information and the recorded data for the accident flight (Recorded flight data), provided that the terrain advisory/alert function was enabled, a yellow and black ‘TERRAIN’ annunciation would be generated in the lower left corner of the LCD display, accompanied by a yellow and black ‘TOO LOW – TERRAIN’ PDA pop-up alert (Figure 10), about 15 seconds prior to the terrain collision.
Figure 10: Premature descent alert on the GNS 430W display
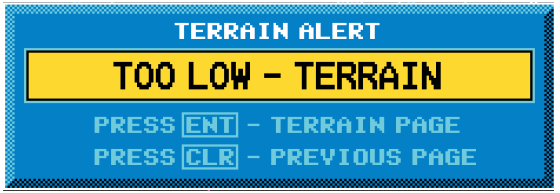
Source: Garmin GNS 400W Series Pilot’s Guide & Reference
The terrain system also provided forward-looking terrain avoidance (FLTA) alerts. Provided the terrain system was enabled, a FLTA terrain alert was generated when the predicted or present aircraft altitude above terrain or obstacles was below the minimum clearance value for that phase of flight. During an approach, the clearance value was 150 ft during level flight and 100 ft when descending.[23] The terrain/obstacle advisory alert comprised a yellow and black ‘TERRAIN’ annunciation in the lower left corner of the LCD display, accompanied by a yellow and black ‘TERRAIN ADVISORY’ or ‘CAUTION OBSTACLE’ pop-up message. The pop-up advisory alert would be displayed on all selectable pages (except the terrain page) and remained visible until the message was cleared/acknowledged by the pilot, or the minimum clearance value was no longer infringed.
If the minimum clearance value for terrain/obstacles remained, a terrain/obstacle ahead alert would be generated. The alert consisted of a flashing yellow and black ‘TERRAIN’ annunciation in the lower left of the LCD panel display and a flashing yellow and black ‘TERRAIN AHEAD’ or ‘OBSTACLE AHEAD’ pop-up alert. The pop-up alert would be displayed on all selectable pages, except the terrain page and remained visible until the message was cleared/acknowledged by the pilot, or the minimum clearance value was no longer infringed.
The GNS 400W-Series manual did not specify the warning period for FLTA alerts. However, an earlier version of the 430/430A-Series manual indicated the ‘TERRAIN ADVISORY’ or ‘OBSTACLE ADVISORY’ pop-up terrain alert would be displayed when approximately 60 seconds from potential impact and the ‘TERRAIN AHEAD’ or ‘OBSTACLE AHEAD’ flashing pop-up terrain alert when 30 seconds from potential impact.
On the accident flight, the descent rate when the aircraft reached 5 NM from the runway threshold (or 3.6 NM from the MAPt) is unclear (see Recorded flight data). However, soon after, the descent rate was about 1,200 ft/min and the predicted flight path would have resulted in an FLTA alert to be generated. Therefore, if the terrain awareness/alert system was enabled, an FLTA alert should have been generated about 30 seconds (or longer) prior to the collision.
Air Connect Australia did not have any operational guidance or procedures regarding the use of the terrain awareness function on the GNS 430W units. The chief pilot reported that, when they were the pilot flying VH-OZO, they would generally leave the function turned on, even though it could be annoying in some locations. However, they did not regularly fly the aircraft and had not conducted the most recent flights in the aircraft.
The ATSB spoke to several pilots who were familiar with GNS 430 units with a terrain awareness function. Some advised that the terrain awareness function would often be inhibited, whereas others would use the function on one 430 unit and inhibit it on the second 430 unit. None of the pilots were aware of operators having specific procedures and guidance for using the terrain function.
Pilots stated that the main reason for the terrain awareness function to be inhibited was the perception that it could issue nuisance alerts, with some of these pilots clarifying that this would only occur (or be a valid concern) when conducting visual approaches at non-licenced aerodromes. One pilot advised that they had heard of some pilots in one operator being concerned about using the terrain function if the terrain/obstacle database was not current. However, 2 experienced flight examiners advised that, in their experience, these databases were commonly not current on aircraft they encountered in their roles and that having a current database was not critical; the advantages of the terrain awareness function were more significant than any potential problem with a terrain/obstacle database not being current.
There was no information available regarding what the pilot of the accident flight normally did with the terrain awareness function when flying VH-OZO. Based on the available information, the ATSB could not establish if the terrain awareness function was enabled or inhibited during the accident sequence, or to what extent the pilot had previously encountered terrain alerts when conducting operations in the aircraft.
Configurations and speeds
The flap settings on the Cessna 404 included ‘UP’, ‘T.O. & APPR’ (take-off/approach) and ‘LAND’ (landing). The take-off/approach setting was often referred to as ‘approach flap’ and sometimes called ‘10° flap’.[24]
According to the Cessna 404 Pilot’s Operating Handbook (POH), which included the Airplane Flight Manual approved by the US Federal Aviation Administration, the maximum landing gear extension speed (and operating speed) was 182 kt, the maximum speed to select approach flap was 182 kt, and the maximum speed to select landing flap was 152 kt.
The POH specified a recommended minimum approach speed (or Vref[25]) at 50 ft of 91 kt (all engines operating, landing flaps, weight 8,100 lb or 3,6764 kg). For all aircraft weights of 7,500 lb (3,402 kg) and lower, the POH stated approach airspeeds (at 50 ft) of 88 kt. Consistent with the POH, the operator’s operations manual provided values for VAPP[26](approach speed) of 91 kt at 8,100 lb and 88 kt at 7,500 lb and lower weights.
The POH stated the minimum control speed (VMCA) with approach flap selected was 78 kt. In addition, the one-engine inoperative best rate-of-climb speed for the aircraft type was 102 kt (flap in the take-off/approach position and gear up) and 109 kt (flap and gear up).
Aircraft maintenance
The aircraft logbook statement specified that VH-OZO was to be maintained in accordance with the system of maintenance developed by the aircraft owner and approved by CASA. The key elements of the system were:
- daily inspection in accordance with the Cessna 404 POH
- engine and airframe inspections every 100 +/- 10 hours in accordance with the Cessna 404 progressive care program (Operations 1 and 2 plus 3 and 4 completed within 12-month period)
- electrical and instrument inspections every 220 hours or 12 months in accordance with system of maintenance schedules
- IFR avionics inspections every 220 hours or 12 months in accordance with system of maintenance schedules
- special inspections, supplemental inspection documents, and corrosion prevention control program as required
- altimeter and pitot-static system inspection and test every 24 months
- maintenance release issue for a period of up to 220 hours or 12 months, whichever occurred first.
Scheduled engine and airframe maintenance was carried out by the CASA-approved maintenance organisation associated with the aircraft owner. While the aircraft was based in Cairns, electrical, instrument, and radio maintenance as well as unscheduled maintenance was contracted to licensed aircraft maintenance engineers.
The most recent maintenance was the scheduled 100-hour inspection based on Operations 3 and 4 of the Cessna 404 progressive care program. That was completed on 16 February 2020 at 31,066 hours total time. A maintenance release was issued with the next scheduled maintenance being the oil/filter change after 50 hours operation and compass swing in July 2020.
Other key maintenance was:
- 19 January 2020 at 31,050 hours: inspection of the electrical, instrument and IFR avionic systems certified as satisfactory
- 29 January 2019 at 30,750 hours: inspection and test of the pitot-static system and check of altimeters certified as satisfactory.
The current maintenance release was not found at the accident site. Operator records showed that the aircraft had been operated for 3.8 hours between maintenance release issue and the accident flight. The operator and aircraft owner both advised that no aircraft defects had been reported.
The ATSB identified that the vacuum pumps had been in service for a relatively long period and internal wear had not been inspected at the recent 100-hour inspection. Also, there was no record of testing or replacement of the vacuum manifold in the previous 10 years. Although these aspects increased the likelihood of a vacuum system failure, there was no evidence that the vacuum-powered instruments were adversely affected. Further information regarding the maintenance and serviceability of the vacuum system (associated with the attitude indicators and directional gyro) is provided in Appendix B – Vacuum system analysis.
Terrain avoidance and warning systems
General description
A terrain avoidance and warning system (TAWS) provides visual and aural alerting including a look-ahead terrain function. The aircraft’s height above terrain can be based on GPS or radio altitude information. TAWS is a generic term that also includes a ground proximity warning system (GPWS) with a forward-looking terrain avoidance function.
A TAWS is an important tool to help minimise the risk of controlled flight into terrain (CFIT). It provides an independent and unambiguous warning of proximity to the ground or obstacles, regardless of any navigational uncertainty or error such as mis-setting or misreading the altimeter.
Multiple levels or classes of TAWS are defined and internationally recognised. Class B TAWS (TAWS B) includes a minimum of the following alerts:
- reduced required terrain clearance
- imminent terrain impact
- premature descent
- excessive rates of descent
- negative climb rate or altitude loss after take-off
- descent of the aeroplane to 500 ft above the terrain or nearest runway elevation (voice callout ‘Five hundred’) during a non-precision approach.
Class B+ TAWS also has a terrain awareness display that shows surrounding terrain/obstacles relative to the aircraft. Class A TAWS (TAWS A) has all the requirements of Class B+ TAWS, plus 3 additional alerts. Both Class A and class B TAWS have a forward-looking terrain avoidance function.
To maximise its effectiveness, an aircraft operator should have standard operating procedures for the use of a TAWS and for actions to take in response to TAWS alerts.
Australian requirements
Civil Aviation Order (CAO) 20.18 (Aircraft equipment — basic operational requirements), which was in force at the time of the accident, stated that for Australian aircraft:
9.1C A turbine-engined aeroplane that:
(a) has a maximum take-off weight [MTOW] of more than 15 000 kg or is carrying 10 or more passengers; and
(b) is engaged in RPT [regular passenger transport], or charter, operations;
must not be operated under the I.F.R. unless it is fitted with
(c) an approved ground proximity warning system that has a predictive terrain hazard warning function…
(e) if the aeroplane has a maximum take-off weight of 5 700 kg or less, but is carrying 10 or more passengers – a TAWS-B+ system.
In effect, this meant that turbine-engine aeroplanes being used to conduct passenger transport operations under the IFR were required to have a TAWS if the aeroplane was carrying 10 or more passengers or it was a larger air transport aeroplane.[27] There was no requirement for a piston-engine aeroplane (such as VH-OZO) to be fitted with a TAWS.
International requirements for turbine-engine aeroplanes
The Australian TAWS requirements for turbine-engine aeroplanes were consistent with the standards included in International Civil Aviation Organization (ICAO) Annex 6 (Operation of Aircraft) Part I (International Commercial Air Transport – Aeroplanes), which included a standard[28] for all turbine-engine aeroplanes with a MTOW of more than 5,700 kg or authorised to carry 10 or more passengers to have a TAWS. In addition to Australia, this standard had been adopted by comparable countries, including the United States, Canada, New Zealand and Europe.
In 1996, the US National Transport Safety Board (NTSB) issued a recommendation to the US Federal Aviation Administration (FAA) to require that all turbojet-powered airplanes equipped with 6 or more passenger seats have an operating GPWS installed. In 1999 it also recommended that all turbine-powered aeroplanes of the same size be fitted with a TAWS.
In response, the FAA commissioned a report that examined 44 CFIT accidents that occurred between 1985 and 1994 in the US involving turbine-powered aeroplanes with 6 to 10 passenger seats. Of the 44 aeroplanes, 11 were powered by turbojets and 33 were powered by turboprops. None were fitted with a GPWS system. Computer modelling techniques used to analyse the data showed that, had GPWS been fitted, 33 accidents could have been prevented; had enhanced GPWS been fitted, 42 accidents could have been prevented.
Accordingly, the FAA introduced a requirement for all turbine-powered aeroplanes with 6 or more passenger seats to be fitted with a TAWS B (in Federal Aviation Regulations 91.223 and 135.154). The requirement commenced in March 2002 for new aircraft and March 2005 for older aircraft.
The FAA did not propose to introduce the same requirement for piston-engine (or reciprocating-engine) aeroplanes. In its final rule summary in 2000, the FAA stated:
The General Aviation Manufacturers Association (GAMA) is against requiring TAWS on reciprocating-powered [piston-engine] airplanes because the costs would be high (e.g., “TAWS equipment would cost more than the hull value of the aircraft”), and the panel space for installing TAWS with a situational display is not available in these airplanes.
The FAA did not receive any comments that would justify undertaking a new rulemaking project to mandate TAWS for reciprocating-powered airplanes.
However, regarding the issue of panel space, the FAA knows of at least one manufacturer who has developed a complete TAWS unit that was designed to replace an existing panel instrument.
Following the US introduction, from November 2006, ICAO Annex 6 Part I included a recommendation that turbine-engine aeroplanes with an MTOW of 5,700 kg or less and authorised to carry 6–9 passengers should be equipped with a TAWS. This recommended practice was introduced as a regulatory requirement in other countries, including New Zealand (from 2007 for air transport operations under the IFR), Canada (from 2014 for operations other than day VFR flights), and in Europe (for such aeroplanes with an individual certificate of airworthiness issued after 1 January 2019).[29]
International requirements for piston-engine aeroplanes
Applicable from January 2007, ICAO Annex 6 Part I included a standard for TAWS B to be fitted to piston-engine aeroplanes with a MTOW greater than 5,700 kg or authorised to carry 10 or more passengers.
Subsequently, TAWS requirements were introduced in Canada (from 2014 for air transport operations other than day VFR flights), New Zealand (from 2007 for air transport operations under the IFR) and Europe (from 2012 for air transport operations) for piston-engine aeroplanes. No such requirements for piston-engine aeroplanes were introduced in the United States.
Canada also introduced the same requirement for piston-engine aeroplanes with a passenger seating capacity of 6–9 conducting air transport operations other than day VFR flights from 2014. As far as could be determined, no other countries had introduced a TAWS requirement for piston-engine aeroplanes with a passenger seating capacity less than 10.
In its notice of amendments about TAWS requirements in 2012, Transport Canada indicated that the cost for installing a TAWS B on small aeroplanes conducting air taxi (charter) operations in aircraft with a passenger seating capacity of less than 10 was about Can$23,000. It also noted that the expected benefits of TAWS (in terms of reduced fatalities, serious injuries and accidents due to CFITs) were significantly higher than the costs.[30]
Considerations of changes in Australia
In March 2006, the ATSB issued safety recommendation R20060008:
The Australian Transport Safety Bureau recommends that the Civil Aviation Safety Authority review the requirements for Terrain Awareness Warning Systems for Australian registered turbine-powered aircraft below 5,700 kgs, against international standards such as ICAO Annex 6 and regulations such as FAR 91.223, with the aim of reducing the potential for CFIT accidents.
CASA accepted the recommendation and stated that it would examine the capital/installation costs and benefits. This work was initiated as part of CASA’s notice of proposed rule making (NPRM) 0808OS (Passenger transport services and international cargo operations – Small aeroplanes) published in February 2009. The proposed requirements included that small aeroplanes (regardless of engine type) conducting passenger transport operations carrying 6 or more passengers under the IFR to be fitted with a TAWS B. In terms of benefits and costs, the NPRM stated:
The costs and benefits of mandating TAWS B equipment for IFR aeroplanes carrying 6 to 9 passengers has been assessed by CASA. Equipment and fitment costs are forecast to be approximately $23,000 per aeroplane. Options to offset these additional costs are under consideration by the Government. Benefits are expected to flow to the industry from increased public confidence with this equipment fit to small aeroplanes in which passenger operations are conducted, as the overall accident rate is expected to reduce.
Accordingly, the ATSB recommendation was closed.
Subsequently, CASA released a consultation draft of Civil Aviation Safety Regulation (CASR) Part 135 (Australian air transport operations—smaller aeroplanes) in 2012. This contained a requirement for a TAWS for aeroplanes conducting passenger transport operations with a maximum operational passenger seat configuration (MOPSC)[31] of 6 or more. The proposed requirement’s applicability had expanded to include all flights (not just IFR flights) and was based on the passenger seat capacity rather the actual number of passengers carried on a flight.
Following further consultation, the 2012 proposal was amended to a MOPSC of 10 or more. In the summary of proposed change for CASR Part 135 (published in August 2018), CASA stated:
CASA had originally proposed the fitment of a minimum of TAWS-Class B for aeroplanes with a MOPSC greater than 5 which aligned with the Federal Aviation Administration of the USA (FAA) and Transport Canada rules however this was changed after discussion with the Aviation Safety Advisory Panel Technical Working Group.
In the 2018 explanatory statement in for the introduction of CASR Part 135, CASA outlined the options it considered (in its regulatory impact statement) regarding the implementation of TAWS. It noted that the estimated cost of installing a TAWS was about $21,000 (including about $12,000 for the system and additional costs for installation and training). One option (named option 2) was to only require a TAWS for all aeroplanes with a MOPSC of 10 or more or a MTOW greater than 5,700 kg. It was determined that this would affect 18 aeroplanes (in addition to those that already had or required a TAWS). Another option (option 3) was to require a TAWS for all aeroplanes with a MOPSC of 6–9 as well. In terms of option 3, the statement noted:
The types of aircraft that are within this category include, the piston powered AeroCommander 680, Beech 95 and Cessna 421 and the turbine powered aeroplanes that include the Cessna 208, Fairchild SA 226 and Pilatus PC 12. CASA estimates that there are approximately 323 of these types of aircraft. Based on 323 aircraft within the six to nine seat range and the 18 aircraft with MTOW>5700kg of option 2 this would result in an estimated cost impact of $7.2m for 341 aircraft... [as well as $0.72m annually]
The statement noted that initially CASA had proposed option 3 to industry but, following initial consultation, it did not pursue this option. Feedback associated with the initial consultation on TAWS (and other proposed regulatory changes) included that charter businesses were operating in a difficult marketplace with many not being profitable.
As a result of this regulatory reform process, the Australian requirements for TAWS changed from December 2021, such that piston-engine aeroplanes with a MOPSC of 10 or more conducting air transport operations were required to have a TAWS, with the applicable date being December 2021 or December 2022 dependent on various factors (see Safety issues and actions).
For VH-OZO and its seating configuration at the time of the accident, CASA advised the MOPSC was 12.[32]As such, if the operator had continued operating the aircraft for passenger transport flights with that seating configuration, the aeroplane would have been required to have a TAWS by December 2022 and, from December 2024, the operator would have had to operate the aeroplane under CASR Part 121 (and conduct all flights with 2 pilots under the IFR, as well as meet additional requirements compared to Part 135). Alternatively, the aeroplane would not have been required to have a TAWS or be operated under Part 121 if some seats were removed such that the MOPSC was 9 or less.[33]
Aerodrome information
Lockhart River Airport had one sealed runway (12/30), which was 1,500 m long and 30 m wide. It was not serviced by an air traffic control (ATC) tower and it was outside of ATC radar coverage. The airport had a common traffic advisory frequency (CTAF), which was used by pilots to advise intentions and arrange separation with other traffic.
The elevation of the runway was 76 ft at the threshold for runway 30 and 48 ft at the threshold for runway 12. The runway was equipped with low-intensity runway lights, and there was no visual approach slope guidance.
At the time of the accident there were 3 instrument approaches available at the airport:
- NDB approach to runway 30
- RNAV GNSS approach to runway 12
- RNAV GNSS instrument approach to runway 30 (Figure 2).
The airport was located on a coastal plain 4.5 km west of the Lockhart River township. The Great Dividing Range was nearby with the terrain rising to over 800 ft to the south-west and west within about 8 km of the airport.
Lockhart River was known to experience low cloud and poor visibility conditions. The average (mean) rainfall at Lockhart River is 2,058 mm. In the month of March, the average rainfall is 446 mm and 19.5 days have rainfall of more than 1 mm.
The ATSB interviewed several pilots with experience conducting RNAV GNSS approaches, including some who had conducted multiple approaches at Lockhart River due to poor visibility conditions. The pilots had several suggestions for reducing workload for a second approach, including holding or diverting to Coen or Weipa to wait for weather to pass.
Meteorological information
Weather forecasts - overview
The Bureau of Meteorology produced aviation forecasts, observations, warnings and advisories. As the official provider of the Aeronautical Information Service, Airservices Australia delivered the bureau’s aviation meteorological products to pilots through National Aeronautical Information Processing System (NAIPS).
For the flight from Cairns to Lockhart River, the meteorological forecast information consisted of aerodrome forecasts (TAFs), graphical area forecasts (GAFs), grid point wind and temperature charts (GPWTs) and any warnings (such as SIGMETs[34]). These could be supplemented by aerodrome weather reports (METARs), ground-based weather radar imagery, and satellite imagery.
According to the weather forecasts, the pilot would have expected mostly visual meteorological conditions (VMC)[35] during the day at Cairns with some periods of rain showers and low cloud. For the arrival at Lockhart River, the forecast weather was predominantly VMC but there were overlapping periods of rain and low cloud with 30% probability of thunderstorms.
Aerodrome forecasts
A TAF for Lockhart River was issued at 0449 EST[36] and was valid from 0600 to 1800. The expected weather conditions were:
- From 0600 to 1000: wind variable at 3 kt with visibility 10 km or greater. Light rain showers and cloud scattered at 1,000 ft.[37]
- Between 0600 and 1000: TEMPO[38] - visibility reduced to 3,000 m with rain and broken cloud at 500 ft.
- From 0600 to 0800: 30% probability of fog with visibility reduced to 500 ft and broken cloud at 100 ft.
- From 1000 to 1800: wind from the north-east at 5 kt with visibility 10 km or greater. Light rain showers with scattered cloud at 1,000 ft.
- Between 1000 and 1800: TEMPO - visibility reduced to 3,000 m with rain showers and broken cloud at 800 ft.
- For the whole forecast period, 0600 to 1800: 30% probability TEMPO - winds gusting 25 to 35 kt and visibility reduced to 1,000 m due to thunderstorms and rain. This was associated with broken cloud at 500 ft and scattered cumulonimbus cloud with the base at 1,000 ft.
Based on this forecast a pilot arriving at Lockhart River was required to plan for 60 minutes holding or diversion to an alternate aerodrome. The aircraft had more than sufficient fuel for that purpose (see Fuel calculations).
An amended TAF for Lockhart River was issued at 0925 (5 minutes after the accident) and was valid from 0900 to 1800. The expected weather conditions were:
- From 0900 to 1300: wind variable at 3 kt with visibility 10 km or greater. Light rain showers with cloud scattered at 1,000 ft and broken at 2,000 ft.
- For whole forecast period, 0900 to 1800: TEMPO – winds gusting from 20 to 35 kt and visibility reduced to 1,000 m due to thunderstorms and rain. This was associated with broken cloud at 500 ft and scattered cumulonimbus cloud with the base at 1,500 ft.
Graphical area forecasts
A GAF was issued at 0835 and was valid from 0900 to 1500 and applicable from surface to 10,000 ft. This covered the Queensland-North region, which was divided into 6 areas for this forecast. Most of the flight including the arrival at Lockhart River was within one area that was forecast to have the following conditions:
- Broken stratus 1,000 ft to 2,000 ft with broken cumulus/stratocumulus above that. Visibility reduced to 6,000 m in widespread rain.
- Isolated towering cumulus from 2,000 ft, broken stratus from 800 to 2,000 ft, and broken cumulus/stratocumulus from 2,000 ft. Visibility reduced to 2,000 m in scattered rain showers.
- Isolated cumulonimbus from 2,000 ft and broken status between 500 ft and 1,000 ft. Visibility reduced to 500 m in isolated thunderstorm rain showers.
A GPWT forecast was issued at 0538 and was valid to 1000. Lockhart River was located near the intersection of 4 data boxes and therefore roughly equidistant from 4 forecast locations. Taking 2,000 ft as a reference height for the approaches and coastal data as more relevant, the wind was forecast to be from the north-west at 9 kt increasing to 21 kt north of Lockhart River.
There were no significant weather warnings applicable to the flight.
Weather conditions - overview
The Bureau of Meteorology provided an overview to the ATSB of the actual weather conditions at Lockhart River on the day of the accident. It stated that a developing monsoon trough extended across Cape York Peninsula crossing the coast near Weipa and Lockhart River. A tropical low was embedded in the trough and was near Weipa at 1000, moving slowly eastward. The monsoon trough and low were causing scattered to widespread rain and isolated thunderstorms over much of Cape York Peninsula.
The surface winds at Lockhart River Airport were generally light and variable. Automatic weather sensors detected rain and heavy rain reducing visibility to 800 m and scattered to broken layers of cloud as low as 1,100 ft above ground level.
Aerodrome weather reports for Lockhart River
The METARs for Lockhart River were automatically generated every 30 minutes for routine reports and were issued as a special report (SPECI) at other times when one or more elements met specified criteria for degradation and improvement. For the period from 0830 to 0929 on 11 March 2020, the reports included:
- 0830: nil wind, visibility[39] 10 km or greater with rain and scattered cloud from 3,000 ft. Temperature and dewpoint were both 25 °C. Rainfall in the previous 10 minutes was 0.4 mm.
- 0900: nil wind, visibility 10 km or greater with rain and broken cloud at 2,000 ft, 3,500 ft, and 4,100 ft. Temperature and dewpoint were both 25 °C. Rainfall in the previous 10 minutes was 0.4 mm.
- SPECI 0910: nil wind, visibility 10 km or greater with rain and broken cloud at 1,800 ft and 3,400 ft then overcast at 4,200 ft. Temperature and dewpoint were 26 and 25 °C respectively. Rainfall in the previous 10 minutes was 0.4 mm.
- SPECI 0913: nil wind, visibility 3,800 m with rain and broken cloud at 1,800 ft and 3,400 ft, overcast at 4,200 ft. Temperature and dewpoint were 26 and 25 °C respectively. Rainfall in the previous 10 minutes was 2.2 mm.
- SPECI 0929: wind westerly at 5 kt, visibility 8,000 m with heavy rain and scattered cloud at 1,200 ft, broken cloud at 1,900 ft, and broken cloud at 3,600 ft. Temperature and dewpoint were both 25 °C. Rainfall in the previous 10 minutes was 0.4 mm and rainfall since 0900 was 3.8 mm.
The QNH remained at 1,008 hPa during this period.
Automatic weather station
The Bureau of Meteorology (BoM) provided data from the Lockhart River Airport automatic weather station (AWS) recorded at 1-minute intervals. Table 3 details 1-minute rainfall, 30-minute cloud and 10-minute visibility data for the period encompassing the aircraft’s first approach prior to the final approach fix (FAF) at 0904 to the accident at 0920. The last column in the table indicates the 1-minute visibility associated with the telephone message for the aerodrome’s automated weather information service (see following section section). More detailed information from the Lockhart River AWS for the period 0850 to 0930 is provided in Appendix C – Detailed 1-minute weather data Lockhart River 0850–0930.
Table 3: Extract of 1-minute weather data from Lockhart River Airport AWS
Time (EST) | 1-minute precipitation (mm) | Lowest cloud layer amount (oktas) | Lowest cloud layer height above aerodrome elevation (ft) | 10-minute visibility (m) | 1-minute visibility reported by AWIS (m) |
0904 | 0.2 | 2 (few) | 1,800 | 10,000 | 10,000 |
0905 | 0.0 | 2 (few) | 1,800 | 10,000 | 10,000 |
0906 | 0.0 | 3 (scattered) | 1,800 | 10,000 | 10,000 |
0907 | 0.0 | 3 (scattered) | 1,800 | 10,000 | 10,000 |
0908 | 0.0 | 3 (scattered) | 1,800 | 10,000 | 10,000 |
0909 | 0.0 | 4 (scattered) | 1,800 | 10.000 | 12,000 |
0910 | 0.2 | 4 (scattered) | 1,800 | 10.000 | 6,000 |
0911 | 0.2 | 5 (broken) | 1,800 | 10,000 | 1,500 |
0912 | 0.8 | 5 (broken) | 1,800 | 6,000 | 800 |
0913 | 0.8 | 5 (broken) | 1,800 | 3,800 | 1,000 |
0914 | 0.4 | 5 (broken) | 1,800 | 2,800 | 1,400 |
0915 | 0.2 | 5 (broken) | 1,800 | 2,400 | 2,300 |
0916 | 0.2 | 4 (scattered) | 1,800 | 2,200 | 4,300 |
0917 | 0.0 | 4 (scattered) | 1,800 | 2,200 | 3,600 |
0918 | 0.2 | 4 (scattered) | 1,800 | 2,100 | 4,800 |
0919 | 0.2 | 3 (scattered) | 1,800 | 2,000 | 4,800 |
0920 | 0.0 | 3 (scattered) | 1,800 | 1,900 | 6,000 |
0921 | 0.0 | 2 (scattered) | 1,800 | 2,000 | 8,000 |
The first shaded area indicates when the aircraft was at the MAPt on the first approach and the second shaded area indicates the time of the accident on the second approach. The 1-minute visibility data was that recorded to be broadcast by the AWIS by telephone. The times have been displaced by 1 minute (back) to align with the 1-minute rainfall data provided by BoM. The cloud data was averaged over the previous 30 minutes (see description in report text).
Source: Bureau of Meteorology
The cloud information was derived using a sky condition algorithm. This involved taking a sample (using a ceilometer at the aerodrome) at least every 30 seconds and averaging the data over a 30-minute period, with samples taken in the last 10 minutes provided double weighting.[40] Although this algorithm provided more stable estimates of cloud than each sample, the reported cloud for any given minute did not necessarily reflect the cloud level at that specific point in time.[41]
The visibility meter estimated atmospheric visibility based on the continuous sampling of a single point, providing a measurement of the prevailing visibility at the sensor. However, it sampled a relatively small volume of air in the immediate vicinity of the instrument and, unlike a human observer, was not capable of estimating visibility in different directions or over longer distances. One-minute data was based on the last 60 seconds of sensor output, as an average based on a processing algorithm. The 10-minute data recorded each minute was the average value over the previous 10 minutes.
A number of factors can affect the accuracy of the reported visibility, including:
- discrete air masses, such as a shower of rain or a bank of fog, will not be identified unless the sensor is engulfed, and if the phenomenon is not of uniform density the visibility will be misreported
- stationary localised patches of fog will remain undetected if the sensor is clear of fog, or if the sensor is within the patch of fog the reported visibility may be less than actual.
The temperature and dewpoint were about 25 °C for the duration with the relative humidity at 99% (increasing to 100% from 0917).
The reported wind speeds and directions were the mean values at 10 m above ground level, averaged over the last 1-minute period. The recorded wind was 0 kt until 0914, with speeds of 3–4 kt from the west, between 0916 and 0921.
Automated weather information service
Lockhart River was equipped with an automated weather information service (AWIS) that transmitted AWS data in text-to-speech format on a discrete VHF frequency. A new AWIS message was generated every minute in a similar format to the METAR reports. To produce the reports from the AWS 1-minute data, some averaging and rounding of the information occurred. The broadcasts were not recorded.
In addition, AWIS data at Lockhart River could also be accessed by telephone. A different phone message based on the AWS data was produced every minute and this was recorded. However, the AWIS information available by telephone was processed differently to the AWIS information available by VHF, and therefore there could be slight differences in the information produced at the same time.
During the flight, the pilot recorded the following data in the space allocated for arrival weather information in the flight plan/log:
- calm (nil wind)
- 10 km (visibility)
- B1800 (broken cloud at 1,800 ft)
- B3500 (broken cloud at 3,500 ft)
- OV 5300 (overcast cloud at 5,300 ft)
- 1008 (QNH 1,008 hPa)
- 25 (temperature 25 °C).
This information closely matched the recorded AWS 1-minute data at 0854, which stated (with expected transmitted message in brackets):
- wind 0 kt at 023° (wind calm)
- visibility 10 km (visibility one zero)
- (present weather – rain)
- cloud 4 oktas[42] at 1,800 ft (cloud broken one thousand eight hundred)
- cloud 5 oktas at 3,500 ft (broken three thousand five hundred)
- cloud 7 oktas at 5,300 ft (broken five thousand three hundred)
- temperature 25.4 °C (temperature two five)
- dewpoint 25.2 °C (dewpoint two five)
- QNH 1,008.5 hPa (QNH one zero zero eight hectopascals)
- rainfall last 10 minutes 0.4 mm (rainfall last ten minutes zero decimal four millimetres).
The pilot’s recorded weather information was also broadly consistent with the recorded AWIS messages available by phone for the period 0853–0855. It did not closely match any of the other recorded AWS 1-minute data or the recorded AWIS messages available by phone during 0840–0920. Accordingly, it is very likely that the pilot wrote down the AWIS data accessed by VHF radio at about 0854. It could not be determined whether the pilot additionally accessed the AWIS data prior to this time and/or after this time.
The AWIS broadcasted the most recent 1-minute visibility data measured by the AWS (see Table 3, last column). This data indicated visibility below the landing minima (4,200 m) at the AWS site between 0911 and 0915, and at 0917.
BoM operated a network of weather watch radars around Australia that detected water droplets in the atmosphere. Information from the weather radar was displayed on a map, with different colours depicting the approximate rainfall/precipitation intensity.
The nearest weather radar was at Weipa, approximately 150 km west of Lockhart River. Coverage in the vicinity of Lockhart River was affected by the distance from the radar and terrain, reducing the radar’s ability to detect low-level showers. However, precipitation at higher altitudes would still produce radar echoes.
Figure 11 provides an indication of the weather around Lockhart River at 0918 on the morning of the accident (during the second approach), with Figure 12 displaying an extract of radar images from the time of the first approach and the time of the accident. The weather radar depicted areas of moderate precipitation in the vicinity of Lockhart River. In addition, an analysis of a sequential series of images showed precipitation moving through the area at a speed of about 30 kt (55 km/h) from the north-west.
The minute-by-minute data from the AWS around the time of the accident (see Automatic weather station and Table 3) recorded no significant surface wind. However, there was a short period of rainfall between 0910 and 0916, including moderate to heavy rainfall between 0912 and 0914. If this rainfall persisted and continued moving in a direction/speed consistent with the observed radar returns, it would have been in the vicinity of the accident site about the time of the accident.
Figure 11: Weipa radar image at 0918 EST (about 2 minutes prior to the accident) showing weather in Lockhart River area (circled, approximate radius 25 NM/46 km)
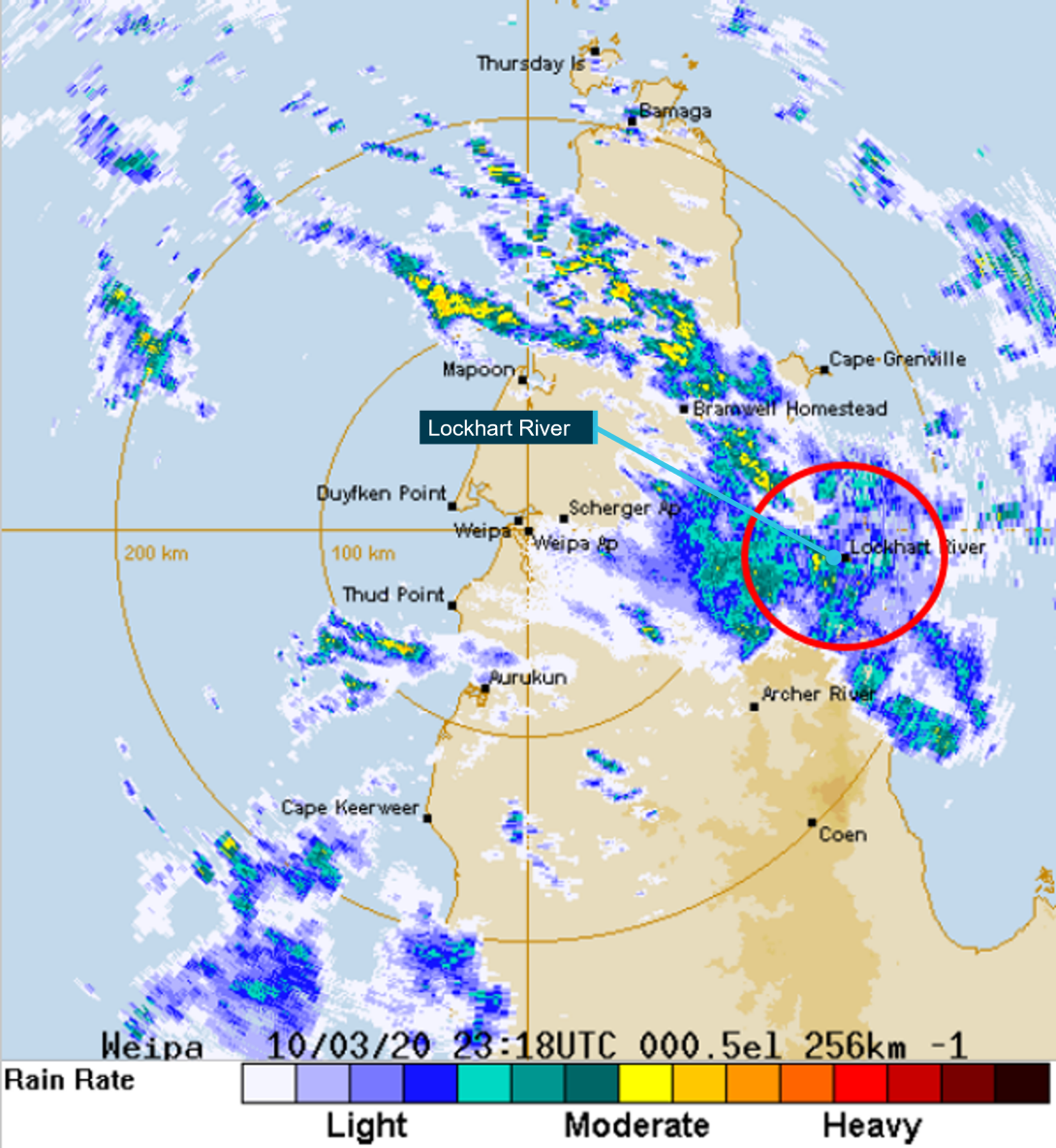
Source: Bureau of Meteorology, annotated by the ATSB
Figure 12: Extract of Weipa radar images at 0906 (time of the first approach) and 0918 EST showing rain rate on the approach path to runway 30 at Lockhart River Airport
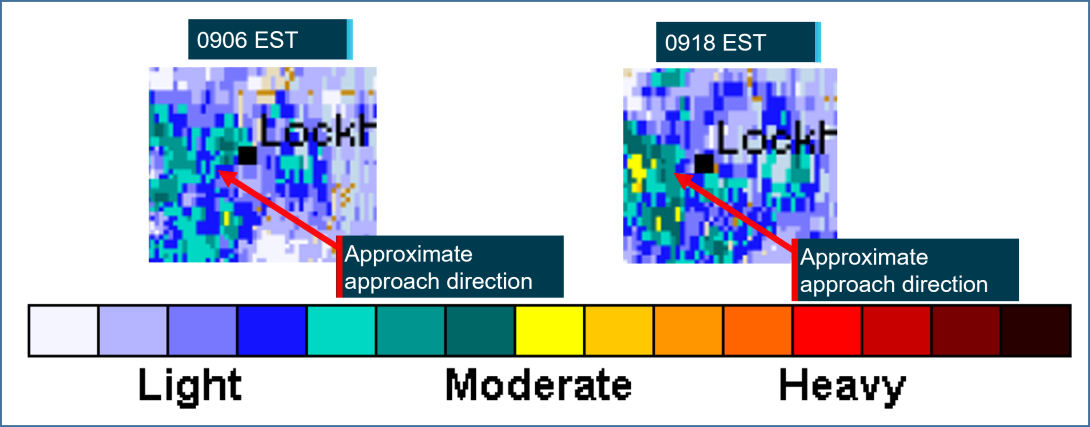
Source: Bureau of Meteorology, annotated by the ATSB
Local weather observations
Two pilots were operating aircraft in the Lockhart River area before and after the accident. The first pilot, operating before the accident, tracked to Lockhart River from the south and conducted the RNAV GNSS runway 30 approach, landing at 0810. There were intermittent rain showers in the area and the pilot advised that the end of the runway was visible while descending through 1,000 ft. The pilot remained on the ground at Lockhart River until later in the day and heard an aircraft (VH-OZO) fly over at high engine power. They recalled that, at that time, there was scattered low cloud at 500–1,000 ft with reduced visibility in rain showers.
The following pilot, operating after the accident, tracked to Lockhart River from the south-west and diverted 15 NM to the right of track due to weather. On arrival, the pilot conducted the Lockhart River RNAV GNSS runway 30 approach and landed at 0953. The pilot reported that there was rain in the area and, although the conditions allowed visual navigation after the final approach fix (FAF) while descending through 1,500 ft, the runway was not visible until later in the approach.
A person who was near the airport at the time of the accident described the conditions as an unusual morning with mist coming from the rainforest, and that there was about 5 to 10 minutes of heavy rain at about the time the aircraft would have been in the area. At that time, there was low-lying cloud (north-west of the airport) and no wind.
Fishermen who were in the area at the time reported that, at about the time of the second approach, there was a ‘wall’ of heavy rain that came across from the north-west.
Instrument approach
Overview
An instrument approach is a published procedure that allows for safe navigation of an aircraft operating in instrument meteorological conditions (IMC) to descend from the lowest safe altitude to a specified position (missed approach point - MAPt) near the aerodrome. If the conditions are suitable, the approach can be continued to land. If the conditions are not suitable, the pilot must conduct a missed approach in accordance with the procedure.
There are 2 general categories of instrument approach:
- 2-dimensional (2D) – lateral/tracking guidance only, also known as non-precision approaches or LNAV (lateral navigation)
- 3-dimensional (3D) – lateral/tracking and vertical guidance, including precision approaches such as an instrument landing system (ILS) approach and a (non-precision) approach with vertical guidance (APV).
Both categories of instrument approaches were only conducted utilising ground-based navigation aids until RNAV GNSS[43] approaches were available in Australia from 1998.
An RNAV GNSS approach is a 2D instrument approach that utilises an on-board GPS receiver (or flight management system - FMS) to generate lateral/tracking guidance and distance information. These approaches are pre-programmed in a GPS/navigation system’s database. Other types of non-precision approaches use ground-based aids such as a non-directional beacon (NDB) or a VHF omni directional radio range (VOR).
The transition in Australia from navigation reliant on ground-based navaids to performance-based navigation is continuing. As part of that process, Airservices Australia has been implementing barometric vertical navigation (Baro-VNAV) APV approaches since 2016. These 3D approaches are restricted to runways with a validated (LNAV/VNAV RNP APCH) procedure and aircraft with the applicable avionics, typically an FMS. Although these approaches were available at Lockhart River from 3 December 2020, VH-OZO was not Baro-VNAV capable.[44]
RNAV GNSS approach design
RNAV GNSS approaches in Australia have a Y-pattern, runway-aligned design. The Lockhart River runway 30 RNAV GNSS approach uses this typical layout, as shown in Figure 13.
Figure 13: Extract from Lockhart River RNAV GNSS runway 30 approach chart showing layout of waypoints
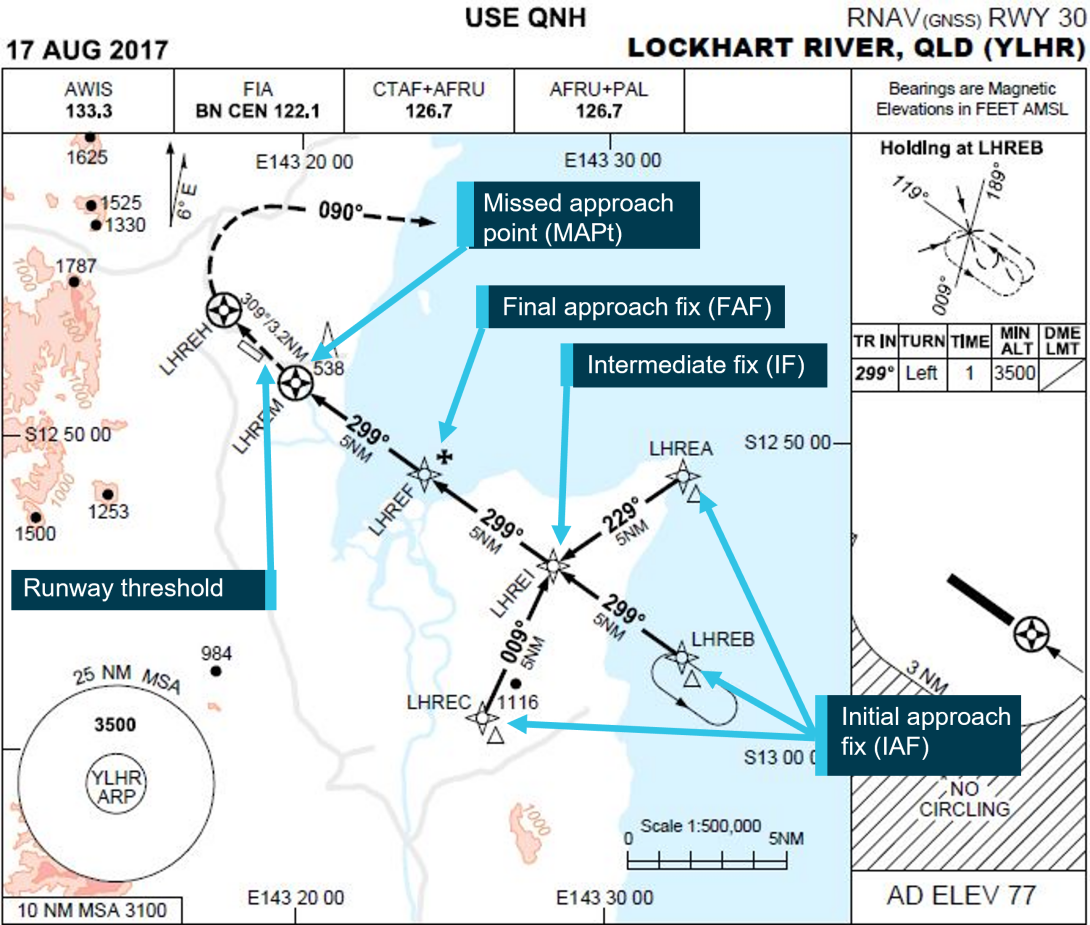
Source: Airservices Australia, annotated by the ATSB
The approaches have 3 initial approach fixes (IAFs, in this case, LHREA, LHREB and LHREC), followed by an intermediate fix (IF, LHREI), final approach fix (FAF, LHREF) and missed approach point (MAPt, LHREM).
The segment between an IAF and the IF is the initial approach segment, the segment between the IF and the FAF is the intermediate approach segment, and the segment between the FAF and the MAPt is the final approach segment. Each segment is typically 5 NM.
The intermediate approach track is normally aligned with the final approach track. One of the IAF waypoints is also aligned with the intermediate/final approach track (in this case, LHREB) and the other IAFs are located 70° off the intermediate/final approach track (LHREAS and LHREC). A pilot can commence an approach by flying to one of the 3 IAFs within the capture region for that waypoint. The capture region is a 140° arc for the IAF on the intermediate/final approach track and a 180° arc for the IAFs not on the intermediate/final approach track (with the extreme points parallel to the intermediate/final approach track).
Instrument approach waypoints can either be fly-by waypoints (which require turn anticipation to allow tangential interception of the next segment) or flyover waypoints (at which the turn is initiated). For RNAV GNSS approaches, the IAFs, IF and FAF are fly-by waypoints and the MAPt is a flyover waypoint.
The approaches are recommended to be flown using a continuous descent angle.
The recommended profile published on approach charts consists of an initial approach altitude which then joins a constant descent to 50 ft above the runway threshold. The approach path angle of the descent is normally (and ideally) 3°. Depending on the approach, the initial approach altitude joins the constant descent profile at about the FAF or earlier.
For example, the approach chart for the Lockhart River runway 30 approach (Figure 14) had an initial approach altitude of 3,500 ft leading to an approach path angle of 3°, which commenced 0.8 NM after the IF (4.2 NM prior to the FAF and 9.2 NM prior to the MAPt).
Figure 14: Excerpt from Lockhart River RNAV (GNSS) runway 30 approach chart showing recommended vertical profile and segment minima safe altitudes
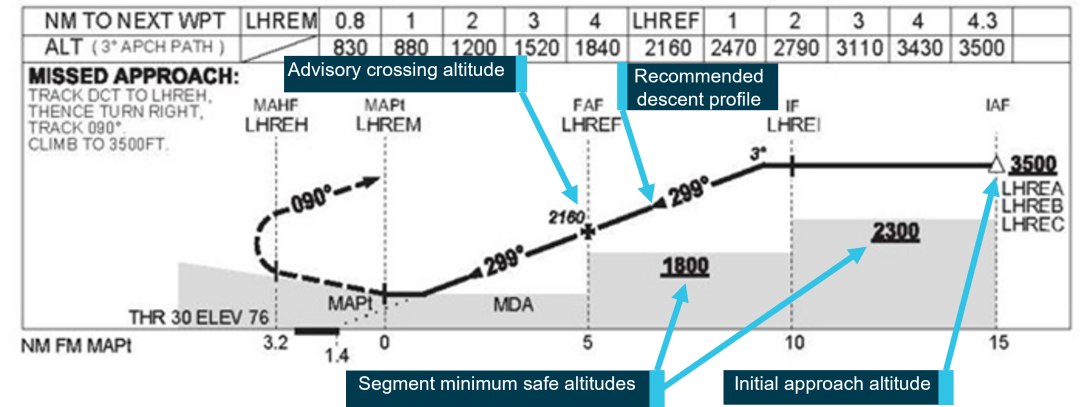
The full approach chart is provided in Figure 2.
Source: Airservices Australia, annotated by the ATSB
Each segment of an RNAV GNSS approach has one or more specified segment minimum safe altitudes, depicted by shading on the chart’s profile diagram and the relevant altitude with a solid line underneath. For example, between the IF and the FAF on the runway 30 approach, the segment minimum safe altitude was 1,800 ft. The last segment minimum safe altitude, in the final approach segment, is the minimum descent altitude (MDA).
During an instrument approach, a pilot is able to descend below the recommended descent profile, but they are not allowed to descend below a segment minimum safe altitude, except for the MDA if certain conditions are met (as discussed below).[45] However, as stated in the Civil Aviation Advisory Publication (CAAP) 178-1(2) (Non-precision approaches (NPA) & approaches with vertical guidance (APV)):
While some pilots in the past have flown NPAs as a series of descending steps conforming to the minimum published altitudes, (a technique colloquially referred to as the ‘dive and drive’), CASA recommends a constant angle descent in a stabilised configuration. Many Controlled Flight into Terrain (CFIT) accidents have been attributed to the ‘dive and drive’ technique, due to human errors such as early descent before a step or failing to arrest descent. In addition, the aircraft’s descent is more difficult to manage due to changes in airspeed, rate of descent and configuration.
If the recommended descent profile starts prior to the FAF, the RNAV GNSS approach chart includes an advisory crossing altitude (or procedure height) at the FAF (and if required the IF) to assist pilots with maintaining the recommended descent profile (for example, 2,160 ft at the FAF in Figure 14). In addition, a pilot is provided with a distance/altitude scale on the approach chart that provides guidance for the recommended descent profile (see top of Figure 14). The distance specified is the distance to the next waypoint.
Approach waypoint naming convention
The waypoints documented on an RNAV GNSS approach chart are identified by a unique identifier comprising the airport identifier (in this case LHR), compass quadrant from which the approach is flown (E - east), and position on the approach (A, B, C, I, F, M or H).
During the investigation, some flight examiners stated that pilots occasionally misidentified waypoints (due to their similar names) and believed that they were on a different segment of the approach than they actually were. One flight examiner noted that this was more likely to occur when coming straight in via the IAF ‘B’ rather than when coming via one of the other 2 IAFs. This flight examiner also noted that one operator they worked with had introduced call-out requirement for pilots to annunciate that they were tracking from one waypoint to another in order to minimise this risk.
Conducting an approach
Before conducting the approach, the pilot must ensure they are qualified for RNAV GNSS approaches and satisfy the recent experience requirements. The aircraft must be equipped with an approved GPS receiver with a valid aeronautical database. It is also essential that the availability of receiver autonomous integrity monitoring (RAIM) is checked pre-flight and before entering the approach.[46]
To conduct the approach, the pilot selects the specific approach and IAF in the GPS unit (or FMS) and tracks towards the applicable IAF within the capture region. Prior to passing the IAF, a pilot must set the QNH to either the actual aerodrome QNH (from an approved source such as AWIS) or the aerodrome/area forecast QNH.
As the approach proceeds, the GPS (or FMS) will automatically sequence through the waypoints, displaying the next waypoint and distance to that waypoint. The pilot maintains the track with reference to the associated course deviation indicator (CDI).
To maintain the recommended descent profile (without electronic vertical guidance), pilots are required to:
- establish a 3° descent by managing the descent rate proportional to the groundspeed (through engine power and pitch angle)
- with reference to the altimeter, monitor the aircraft’s altitude - relative to the distance from the next waypoint with reference to the table on the approach chart and/or when passing waypoints with advisory altitudes
- adjust the descent rate and/or groundspeed as required to correct the profile
- level at the MDA and continue tracking to the MAPt unless the conditions are suitable for continuation of the approach.
The height of the MDA above the aerodrome elevation and the required visibility (in km) to complete the approach were included as bracketed figures after the MDA. If the minima label boxes on the approach chart were shaded, the MDA could be reduced by 100 ft when the actual aerodrome QNH was set. For example, for the accident flight, the pilot had noted the actual QNH so the 830 ft MDA could be reduced to 730 ft (Figure 15). At the lower MDA, the aircraft would be 653 ft above the aerodrome elevation and the minimum required visibility was 4.2 km. Practically, if the visibility was at the minima value, the pilot would see the runway threshold before the MAPt and, if the aircraft was on the recommended profile, by the MDA.
Figure 15: Excerpt from Lockhart River RNAV GNSS runway 30 approach chart showing minima table

The full approach chart is provided in Figure 2.
Source: Airservices Australia
The Aeronautical Information Publication (AIP) outlined several instructions or methods for pilots regarding the conduct of instrument approaches, in addition to those specified by an operator’s operations manual. CAO 20.91 (Instructions and directions for performance-based navigation, Instrument 2014) also applied to the operation of Australian aircraft using performance-based navigation (which included RNAV approaches during IFR flight), providing instructions/directions to operators and pilots conducting those operations. Relevant CAO and AIP requirements, instructions and methods are outlined in subsequent sections below.
Descent below the minimum descent altitude
After passing the FAF, a pilot is permitted to descend to the MDA (provided the aircraft is within navigational tolerances). Further descent below the MDA must not be made without the required visual reference. There may be different MDAs specified for a straight-in or circling approach. Descent below the straight-in approach MDA could only be conducted (AIP ENR 1.5, 1.8.2)[47] when:
- visual reference can be maintained;
- all elements of the meteorological minima are equal to or greater than those published for the aircraft performance category…; and
- the aircraft is continuously in a position from which a descent to a landing on the intended runway can be made at a normal rate of descent using normal flight manoeuvres that will allow touchdown to occur within the touchdown zone of the runway of intended landing.
The AIP also stated that, if visual reference is not established at or before reaching the MAPt, a missed approach must be executed (ENR 1.5, 1.10.1).
The competencies and standards required for an initial instrument rating and IPCs were specified in the CASR Part 61 Manual of Standards (MOS). For a 2D approach, the required tolerance was within +100 ft at the MDA but not below it.
Lateral tolerances
The AIP stated that instrument approach procedures were based on specific navigation aids, ‘with the applicable navigation tolerances associated with the aids being used in the development of the procedure’s obstacle protection surfaces.’ For an RNAV GNSS approach, CAO 20.91 stated that the lateral tracking tolerances were 1.0 NM (1,852 m) for the initial, intermediate and missed approach segments (terminal area operations) and 0.3 NM (556 m) for the final approach segment.
Navigational equipment could be designed so that full-scale deflection on the CDI represented the required navigation performance (RNP) lateral tracking tolerance during that phase of flight. For an RNAV GNSS approach, this would typically be represented as a lateral 1 NM displacement being represented as a full-scale CDI deflection during the initial segment and most of the intermediate segment, then transitioning from about 2 NM (3,704 m) prior to the FAF to a 0.3 NM displacement being full-scale deflection about 1 NM after reaching the FAF (Figure 16).
The GNS 400W-series manual indicated that the CDI full-scale deflection varied during the final approach segment.[48] When conducting an RNAV GNSS approach using a GNS 400W-series unit, the full-scale deflection was 350 ft (0.06 NM or 107 m) at the MAPt, diverging back to the FAF at an angle of either 2° to the final approach track, or an angle such to achieve 0.3 NM full-scale deflection at the FAF, whichever was less (Figure 16).[49] For 5 NM final approach segments, the angular 2° would always be less than the lateral 0.3 NM CDI full-scale deflection at the FAF.
Figure 16: Conventional transition from RNP 1.0 to RNP 0.3 NM during final approach segment (represented by full-scale CDI deflection) compared to the full-scale CDI deflection provided by the GNS 400W-series GPS
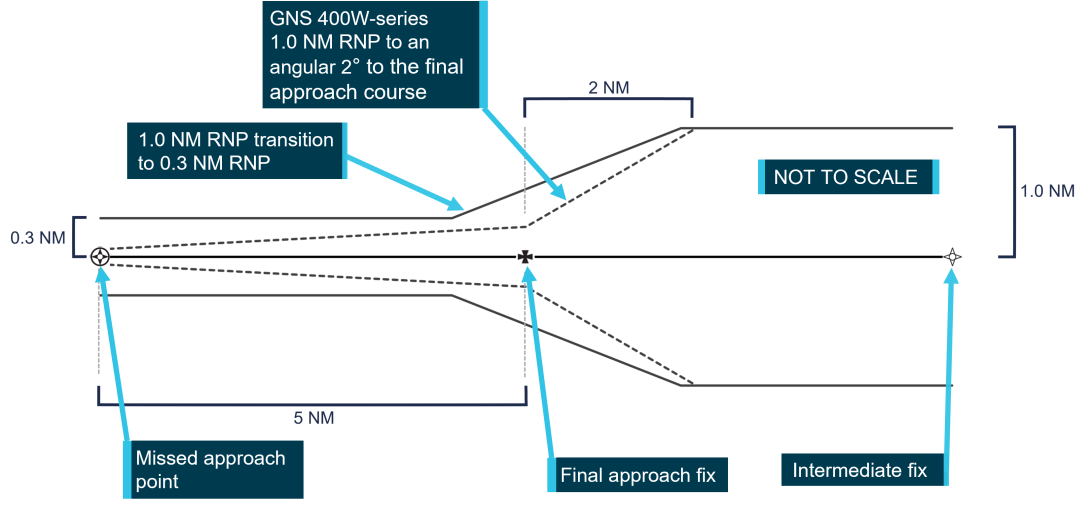
Source: ATSB, derived from information contained in ICAO Doc 8168 Procedures for Air Navigation Services-Aircraft Operations, Vol II − Construction of Visual and Instrument Flight Procedures and the Garmin GNS 400W-Series Pilot’s Guide & Reference manual.
For the 5 NM final approach segment for the runway 30 approach at Lockhart River, an angular 2° to the final approach track displaced 350 ft (107 m) at the MAPt equated to 0.23 NM (430 m) at the FAF. The transition from 1.0 NM full-scale deflection during the intermediate segment to the 2° automatic rescaling full-scale deflection for the final approach segment commenced 2 NM prior to reaching the FAF and was completed by the FAF. In other words, during the final approach segment, GNS 400W series units presented CDI full-scale defection that was less than the lateral tracking limit specified in CAO 20.91 for the final approach segment. This difference was relatively minor at the FAF (0.3 NM versus 0.23 NM) but increased significantly as the aircraft got closer to the MAPt.
When using the GNS 400-series units, if the CDI exceeded a full-scale deflection, a green arrow and distance was displayed on the default navigation page’s CDI, indicating the direction (left or right) and distance the aircraft was displaced (in NM) from the required track.
For a straight-in, area navigation-based approach (such as the RNAV GNSS approach to runway 30 at Lockhart River), the AIP (ENR 1.5, 1.21) stated that an aircraft was required to:
…pass the waypoint, and when established on the specified track, descend to not below the specified altitude….
Note: “Established” means being within half full scale deflection for the ILS, VOR and GNSS... [50]
The AIP (ENR 1.5, 1.10.1) also stated that a missed approach had to be conducted if:
…during the final segment of an instrument approach, the aircraft is not maintained within the applicable navigation tolerance for the aid in use…
CAO 20.91, Appendix 6, contained operating standards for conducting an RNAV GNSS approach, which included the requirement to commence a missed approach if the cross-track error/deviation equalled or was reasonably likely to equal the RNP for that segment of the approach (that is, 1.0 NM for the initial and intermediate segments and 0.3 NM during the final approach segment).
In other words, to comply with the AIP, after passing the FAF, the aircraft needed to be established within half full-scale deflection before descending below the previous segment’s MSA. If the aircraft was established within half full-scale deflection, the descent could continue to an altitude not below the segment minimum safe altitude, which in this case was the MDA.[51] In addition, to comply with CAO 20.91, a pilot was required to conduct a missed approach if the aircraft was laterally displaced 0.3 NM or more from the final approach track.
CASA advised the ATSB that there was, in the aviation community, a commonly held misconception that half full-scale deflection was the required tracking tolerance limit. This was potentially related to a number of factors, such as:
- CAO 20.91 included a note that stated ‘So far as practicable, the cross-track error/deviation for normal operations should be limited to 0.5 NM (½ x RNP) for the initial segment, the intermediate segment and a missed approach, and to 0.15 NM (½ x RNP) for the final approach segment. Brief deviations are acceptable during and immediately after turns where accurate cross-track information is not provided during the turn.’ This reference to half the RNP was providing a target level of safety rather than a minimum acceptable level of safety.
- The CASR Part 61 MOS required tolerance when assessing a pilot’s competency for a 2D approach was within half full-scale deflection.
- CAAP 179A-1(1) (Navigation using the Global Navigation Satellite Systems (GNSS)), issued in 2006, stated (erroneously) that for an RNAV GNSS approach that ‘The tracking tolerance is half of full-scale deflection regardless of the CDI scale’.[52]
CASA advised that operators could choose to specify a more restrictive lateral tracking requirement than that stated in CAO 20.91. If an operator specified a more restrictive limit (such as half RNP or half full-scale deflection), and included that in its operations manual, then that limit would be the applicable limit for that operator.
As discussed in Operator’s stabilised approach criteria, the operator of VH-OZO specified in its operations manual that a missed approach was required if an aircraft on an RNAV approach was not within ‘half scale deflection’ at the FAF. The ATSB is aware through its investigations that a number of other operators also specified a similar requirement for initiation of a missed approach.
Handling speeds
The AIP (ENR 1.5, 1.16) stipulated handling airspeeds for aircraft during instrument approaches based on the aircraft’s performance category. Aircraft performance categories were based on an indicated airspeed at the runway threshold (VAT).[53]
The Cessna 404 VAT was 91 kt. Therefore, the performance category for the Cessna 404 was category B, which applied to aircraft with a VAT of 91–120 kt.
The relevant handling speeds for instrument approaches for category B aircraft were:
- 120 to 180 kt for the initial and intermediate approach segments
- 85 to 130 kt for the final approach segments
- maximum 150 kt for the missed approach.
A note in the AIP stated that a pilot was permitted to reduce the speed below the minimum in the initial/intermediate segments ‘to enable the final approach speed to be achieved prior to the commencement of the final segment’. In other words, for a category B aircraft, a pilot could operate below 120 kt prior to reaching the FAF but they could not operate above 180 kt.
The AIP handling speeds are broad speed bands used for purposes such as ensuring aircraft remain within the design tolerances for instrument approach procedures. Operators should provide more specific guidance regarding the appropriate speeds to use during instrument approaches for their aircraft types (see Flight profiles).
The AIP also stated that the descent rate after the FAF ‘should not normally exceed’ 1,000 ft/min.
Instrument flying and workload
Single pilot IFR operations are widely regarded to be among the most difficult and/or involve the highest workload. As stated by the US Aircraft Owners and Pilots Association (AOPA) in 2006:
No type of flying requires greater skill or longer periods of concentration than [single-pilot IFR] SPIFR…
Very simply, the problem is pilot workload, aggravated by the need for multi-tasking. A single IFR pilot also serves as navigator, radio operator, systems manager, onboard meteorologist, record keeper, and sometimes, flight attendant. En route flight in benign weather is usually not too stressful, but add high-density traffic in poor weather conditions or a significant equipment malfunction, and overload may not be far away.
In instrument flight, the pilot maintains an awareness of the aircraft’s spatial position by reference to instruments rather than outside visual references. The fundamental skills of instrument flight are instrument scanning and instrument interpretation. Instrument scanning is ‘the continuous and logical observation of instruments for attitude and performance information’ (FAA 2012).
In any phase of flight or manoeuvre, there are primary instruments that give the most pertinent information and supporting instruments that assist in their continued correct interpretation. For example, even when the aircraft is established in a constant-rate descent, and trimmed to remove control pressure on the yoke, it is necessary for the pilot to continuously check relevant instruments and make appropriate control adjustments to maintain aircraft performance and control.
With proficiency, a pilot scans at an appropriate rate and is able to interpret the instruments to maintain an accurate mental picture of what is happening. An ineffective scan, such as fixation on one instrument or the omission of another, or misinterpretation of the displayed information, can result in a pilot having an incorrect mental model of the aircraft’s position or flight path.
More generally, workload is described by Wickens and others (2013) as follows:
Mental workload characterizes the demands of tasks imposed on the limited information processing capacity of the brain in much the same way that physical workload characterises the energy demands upon the muscles. In any resource-limited system, the most relevant measure of demand is specified relative to the supply of available resources...
People experience workload differently, based on their individual capabilities and the local conditions at the time such as training and experience in the situation at hand and the operational demands during that phase of flight (Orlady and Orlady 1999). When workload gets too high for the available resources task shedding occurs (Wickens and others 2013).
Dismukes and others (2007) discussed task shedding in this context, explaining that a pilot may move from a proactive assessment of the situation, commonly referred to in aviation as ‘being ahead of the aircraft’, to a reactive situation, where the pilot is responding to events as they occur without an overall strategy to manage the situation (that is, being behind the aircraft). Wickens and others (2013) further explained that task shedding can result in some tasks being shed altogether, and others being shed in a non-optimal manner. In addition, tasks such as internal and external communication are often shed during periods of high workload.
Holmes and others (2003) stated that distractions and high workload can result in a pilot scanning fewer instruments and checking them less frequently. High workload can also lead to a reduction in the number of information sources that an individual will search, as well as the frequency or amount of time these sources are checked (Staal 2004). Additionally, vigilance tasks, such as monitoring flight instruments, require sustained attention with the associated eye movements being fatiguing (Wickens and others 2013).
The United Kingdom Civil Aviation publication, Monitoring Matters, provided information regarding monitoring and stated:
… whilst you are ahead of the game, concentrating on the next event, keeping an eye on all the flight parameters, system modes etc, everything runs fairly smoothly. But as soon as something draws your attention away and you become out of the loop it becomes difficult to play catch up.
Although it is possible to attend to more than one task using selective attention techniques, there is a limit to cognitive capacity. If tasks consume this capacity, that is when task shedding will occur. This publication further advised that under high workload, especially during approach and descent, attention capacity diminishes. This includes the ability to detect when the configuration of the aircraft is not correct, even when there is an aural or visual alert, particularly in the case of single-pilot operations as:
…the processes and procedures will be equivalent to multi crew operation except there will only be one person in the cockpit and the systems may be less automated. Hence the need to monitor the flight profile, flight instruments, fuel state, engines, radios, etc. diligently. The instrument scan must be carried out very frequently, especially during departure and approach in order to monitor the aircraft state and planned profile.
In addition to being a complex skill to acquire, instrument flying skills needs to be maintained by frequent practice (Newman 2007). As stated by CASA in 2016 (Changes to instrument proficiency checks):
Conducting IFR operations is a relatively high risk activity and so requires dedicated knowledge and practical flight training… Skill-based qualifications, like the instrument rating, require the qualification holder to maintain their skills and operational knowledge. Skills and knowledge degrade over time. In the interests of safety, rules are put in place to ensure pilots are sufficiently competent conducting IFR operations.
Accordingly, there are requirements in place for pilots conducting IFR operations to regularly undertake proficiency checks (Proficiency checks and flight reviews) and have recent experience (Monitoring of pilot recency). In general, skill decay or skill degradation increases as the retention interval (or time since learning) increases, and it also increases depending on the quantity and quality of the initial and recurrent training and the amount of task exposure (Arthur and others 1998, Sanli and others 2018, Vlasblom and others 2020). Skill decay has various effects, such as preventing the development of further expertise and decreasing spare mental capacity. As noted by the European Aviation Safety Agency (2021):
Proficiency decay in only a few skills may lead to time management issues, reduced situation awareness, and the ability to keep ahead of the situation. In non-normal situations or emergencies, appropriate actions may not be taken due to one’s inability to analyse the situation as a result of the cognitive overload.
RNAV GNSS approach workload
There has been limited research that has compared different types of instrument approaches, and specifically looked at RNAV GNSS approaches. Research was conducted by the ATSB following a CFIT accident on an RNAV GNSS approach at Lockhart River in 2005 (Godley, 2006). The study found that, for pilots of smaller single-engine and twin-engine aircraft, pilot workload was perceived as being higher, and reported losses of situational awareness were more common, for RNAV GNSS approaches compared to other approach types except for NDB non-precision approaches.
In contrast, pilots of larger aircraft found that RNAV GNSS approaches were not as problematic, with the workload only being higher than ILS (precision) approaches. The different aircraft category responses were likely to have been due to high capacity aircraft having advanced automation capabilities and operating mostly in controlled airspace. Such aircraft were also more likely to have an FMS rather than a GPS unit.
The concern most respondents had regarding the design of RNAV GNSS approaches was that they did not use references for distance to the missed approach point on the approach chart and cockpit displays (in contrast to previous types of approaches). Other problems raised were short and irregular segment distances and multiple minimum segment altitude steps, that the RNAV GNSS approach chart was the most difficult chart to interpret, and that five letter long waypoint names differing only by the last letter can easily be misread. The most common incident reported with RNAV GNSS approaches was commencing the descent too early due to a misinterpretation of position.
It should be noted that at the time of this research, many GPS units displays only provided numerical data rather than a map view of the aircraft’s lateral position (such as with the GNS 430W). In addition, RNAV GNSS approaches have become more common and pilots have become more familiar with them, and the design of RNAV GNSS approach charts has improved.
Recorded flight data
General information
The aircraft was not fitted with a flight data recorder or cockpit voice recorder, nor was it required for the type of aircraft and operation.
The ATSB obtained data broadcast by the automatic dependent surveillance broadcast (ADS-B)[54] equipment fitted to the aircraft and GPS data from the pilot’s iPad with the OzRunways[55] application installed. The data included:
- timestamp
- latitude and longitude
- pressure altitude (from the ADS-B data) – rounded to the nearest 100 ft (for example, 498 ft would be rounded to 500 ft)
- GPS altitude (from OzRunways) – truncated to 100 ft (for example, 498 ft would be presented as 400 ft)
- groundspeed.
The lateral location (latitude and longitude) from OzRunways and ADS-B-based data were in close agreement. This indicated that the data from OzRunways was sufficiently reliable to show the aircraft’s lateral flight path.
The pressure altitude data from the ADS-B data was fairly consistently 100 ft higher than the OzRunways GPS height, which was partially due to the ADS-B data being rounded to the nearest 100 ft and the OzRunways data being truncated to the nearest 100 ft. In addition, the local QNH was 1,008.5 to 1,008.9 hPa during the period from 0900 to 0921, lower than the standard 1,013.25 hPa datum for pressure altitude. This would result in an altimeter set to the local QNH reading about 130–140 ft lower than the pressure altitude.[56] Thus, within the applicable errors, the altitudes from the 2 sources were consistent.
The OzRunways data points were provided at 5-second intervals, with some data points missing, and they covered the whole flight (including all of the 2 approaches), whereas the ADS-B data points were only available for parts of the approaches and/or were provided at less frequent intervals. As such, the OzRunways data is used in this report.
Figure 17 depicts the recorded data for the first and second approaches from the initial approach fix (IAF), through the intermediate fix (IF) and final approach fix (FAF) and to the missed approach point (MAPt). The top panel displays the aircraft’s altitude, the middle panel displays the lateral position, and the bottom panel displays the groundspeed. All distances on the horizontal axes are relative to the next waypoint, as would be displayed to the pilot on the GPS unit during the approach.
Figure 17: Recorded data for first and second approaches to Lockhart River
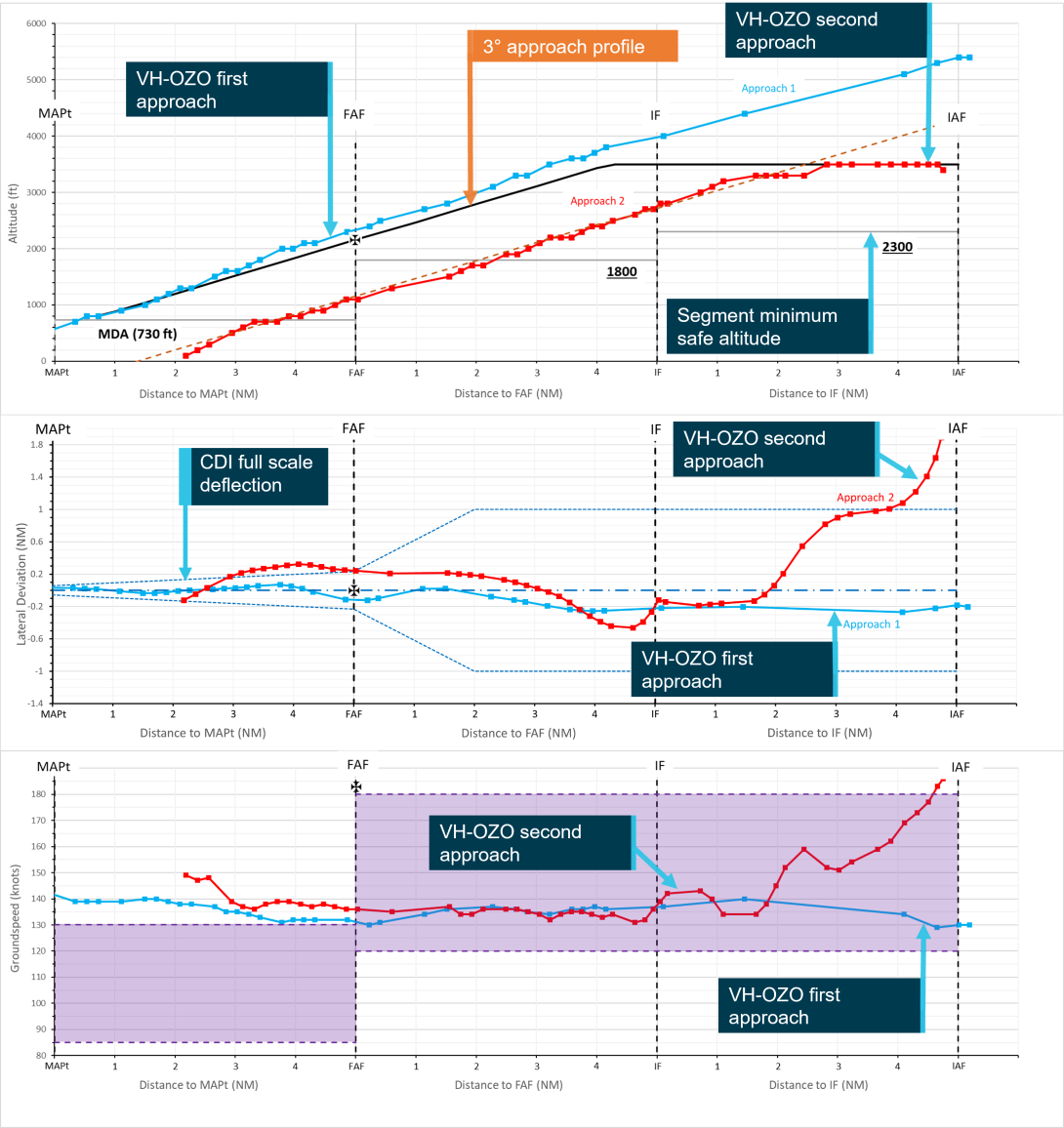
The upper panel shows altitude, the middle panel shows lateral deviation from the instrument approach path and the lower panel shows groundspeed. The purple bands on the groundspeed panel refer to the handling speeds (for indicated airspeed) specified in the Aeronautical Information Publication for a category B aircraft, such as the Cessna 404 (see Handling speeds).
Source: OzRunways data overlaid with Airservices approach information, annotated by the ATSB
Altitude during approaches
The top panel of Figure 17 shows the 3° approach path guidance (recommended descent profile) to the MAPt, which commenced from the initial approach altitude of 3,500 ft about 9.2 NM from the MAPt. As previously noted, the minimum descent altitude (MDA) was 730 ft unless the pilot had the required visibility. Key aspects regarding the recorded altitude data included:
- The first approach (in blue) was flown at or slightly above a 3° approach to the MAPt from significantly prior to the IAF until reaching 700 ft just prior to the MAPt. The average descent rate during the first approach from the IAF to the MAPt was about 720 ft/min.
- Although not indicated on the figure, the aircraft kept descending and reached a recorded altitude of 400 ft[57] at about 0.5 NM past the MAPt or 0.9 NM (1,700 m) before the runway threshold. It remained at that recorded altitude over 3 data points, or until it was 0.5 NM (900 m) from the runway threshold. The next 2 data points were not recorded, with the subsequent recorded data point indicating an altitude of 600 ft as the aircraft passed the runway threshold.
- ADS-B data was available for the latter part of the first approach, and this data indicated that the aircraft had still been descending when it reached the second of the 400-ft data points (0.7 NM or 1,300 m from the runway threshold), and had started climbing just before the third of the 400-ft data points (1,000 m from the threshold). The ADS-B data also provided recorded values of geometric altitude rate of change. This data indicated that just before and just after the MAPt the descent rate was about 900 ft/min, and at about the second 400-ft data point the descent rate was about 960 ft/min.
- The second approach (in red) was flown at about 3,500 ft prior to and after passing the IAF, with the aircraft starting to descend at about 0914:48 when about 2.7 NM from the IF. From about 1.6 NM prior the IF (at 0915:18 and an altitude of 3,300 ft), this descent was flown at about a 3° flight path, although about 1,000 ft below the recommended descent profile.
- The radio call commencing at 0915:50, when the pilot stated the position (10 NM) and altitude of the aircraft (3,800 ft then corrected to 2,800 ft), started about 0.4 NM prior to the IF. At 0915:58, about 0.2 NM prior to the IF, the aircraft was at a recorded height of 2,800 ft.
- For the second approach, the descent rate was about 700 ft/min during the descent from 3,300 ft to about 700 ft (at 0919:08 and 3.3 NM before the MAPt). From 700 ft until 100 ft, the descent rate was about 1,200 ft/min. Given this last part of the descent was over a 30-second period, it probably indicates an actual change rather than the effect of truncated data.
- During both approaches, there were several instances during descent where 2 data points in succession were at the same recorded altitude. There were also 2 occasions during the second approach where 3 data points in succession were at the same recorded altitude (at 700 ft and at 2,200 ft). Although this could indicate that the descent rate decreased to some extent at those altitudes, such patterns could also occur (at least in part) due to the data being truncated to the nearest 100 ft and the small amount of error associated with each GPS data point. A descent rate of about 700 ft/min would equate to about 60-ft difference every 5 seconds, whereas a descent rate of 600 ft/min would equate to about a 50-ft difference every 5 seconds.
Lateral position during approaches
Figure 17 depicts the lateral paths flown on the 2 approaches relative to the lateral track prescribed for the approach (shown as a dash-dot line). Dotted lines depict the full-scale CDI deflection on the Garmin GNS 430W and show the scale transitioning from 1 NM full-scale deflection during the intermediate approach segment to 0.23 NM full-scale deflection at the FAF, narrowing to 0.06 NM full-scale deflection at the MAPt. The aircraft’s position is based on GPS data; the actual CDI values displayed to the pilot were not recorded.
Key aspects regarding the lateral position data include:
- The flight paths for both approaches were consistent with the pilot hand-flying the aircraft, rather than the autopilot maintaining a programmed track.
- The first approach passed the IAF LHREB in the middle of the capture region for that waypoint. The whole approach was contained within half full-scale deflection of the CDI.
- For the second approach, the aircraft was just within the 180° capture region for the IAF LHREB when the pilot commenced the turn towards the IF LHREI (Figure 5). Prior to turning towards the initial approach track, the aircraft was on a track that was about 100° to the initial approach track.
- On the second approach, the turn onto the track between the IAF and the IF resulted in the aircraft initially being displaced full-scale CDI deflection to the right, which was corrected soon after. The aircraft was also turned slightly late at the IF and overshot the waypoint, before being corrected back to the intermediate approach track.
- About 3 NM before the FAF, the aircraft started deviating right of the intermediate approach track. About 2 NM before the FAF, the sensitivity of the CDI began increasing (as indicated by the dotted lines, see also Figure 17).
- When passing the FAF on the second approach, the aircraft was at about full-scale CDI deflection (0.23 NM to the right), and it continued deviating further right of the final approach track for 25 seconds before starting to return closer to the final approach track. The aircraft remained outside full-scale deflection in the final approach segment for about 55 seconds and outside half-full scale deflection for an additional 5 seconds. From about 17 to 32 seconds after passing the FAF, the aircraft’s deviation equalled or slightly exceeded 0.3 NM to the right of the final approach track (the lateral tracking tolerance specified in CAO 20.91 for the final approach track).
- The aircraft started to deviate left of the final approach track at about 2.5 NM prior to the MAPt and it continued left until the end of the recorded data.
Groundspeed during approaches
Deriving an estimate of indicated airspeed from groundspeed involves considering several factors. In this case:
- The recorded wind at ground level indicated nil wind during the first approach and 3–4 kt from about 280° during the second approach. However, a review of wind and temperature forecast and analysis charts from multiple sources indicated that there would have been more wind at higher altitudes. Acknowledging that this was forecast and analysis data rather than recorded data, the ATSB estimated that there would have probably been about 10 kt headwind on the intermediate/final approach track at 2,300 ft and 5 kt at 1,000 ft. In addition, when the aircraft was heading towards the IAF on the second approach at 3,500 ft, and when the aircraft was heading from the IAF to the IF, there would have been a tailwind.
- Air pressure and temperature differences from a standard atmosphere meant that calibrated airspeed would have been lower than the true airspeed, with this difference increasing as the altitude increased. The difference was about 10 kt at 3,500 ft and 5 kt at 1,100 ft.
- The Cessna 404 Pilot’s Operating Handbook (POH) stated that the indicated airspeed at 140 kt was 1 kt higher than the calibrated airspeed with gear and flap up, 3 kt higher with gear down and flap selected to the take-off/approach position, and 5 kt higher with gear down and flap selected to the landing position. During the descent part of an approach, the operator’s flight profile stated the landing gear should be down and the flaps in the approach position (Flight profiles).[58]
Overall, the ATSB estimated that the indicated airspeed would have probably been close to (within 0 to +5 kt) of the recorded groundspeed during the 2 approaches while the aircraft was on or close to the intermediate/final approach track. For simplicity in this report, the groundspeed was considered to be equivalent to the indicated airspeed during these periods.
Key aspects of the recorded groundspeed (and estimated indicated airspeed) data for the 2 approaches include:
- The groundspeed (and estimated indicated airspeed) for the first approach was about 130–140 kt for the whole approach between the IAF and the IF, and 130 kt at the FAF. It then increased to 140 kt before the aircraft reached 1,000 ft and then remained at about that speed as the aircraft passed through the MDA, passed the MAPt and passed the runway threshold.
- For the second approach, the groundspeed when the aircraft was heading toward the IAF was about 185 kt, but the indicated airspeed was about 160 kt. After making the turn towards the IF, the groundspeed was about 160 kt and the indicated airspeed was about 145 kt.
- Before commencing descent from 3,500 ft, at 0914:43, the groundspeed was about 150 kt and the indicated airspeed was about 135 kt. Soon after, there was a decrease in speed, which was probably associated with the pilot selecting approach flap and lowering the landing gear. The subsequent increase was probably associated with the aircraft’s descent.
- Between the IF and the FAF, the groundspeed (and estimated indicated airspeed) was about 135 kt. It increased to 140 kt soon after passing the FAF and, when the aircraft was 3 NM from the MAPt, the groundspeed increased to about 150 kt (associated with the aircraft’s increased descent rate).
Wreckage and impact information
The accident site was located on a sand bank adjacent to the beach, about 6.4 km (3.5 NM) south-east of the runway 30 threshold at Lockhart River Airport and 500 m to the south-west of the specified final approach track. This location was about 2.1 NM (3.9 km) from the missed approach point (MAPt).
The accident site was in line with the aircraft’s recorded track over the previous 3 data points. It was about 200 m beyond the last recorded data point (which had a recorded height of 100 ft), and its location indicated that the impact occurred less than 3 seconds after the last recorded data point.
The wreckage trail was spread over a distance of about 20 m from the initial impact point and the trail indicated that the aircraft was on a heading of about 280° (magnetic), with the impact point about 30 ft above mean sea level (Figure 18).
Figure 18: Overview of accident site
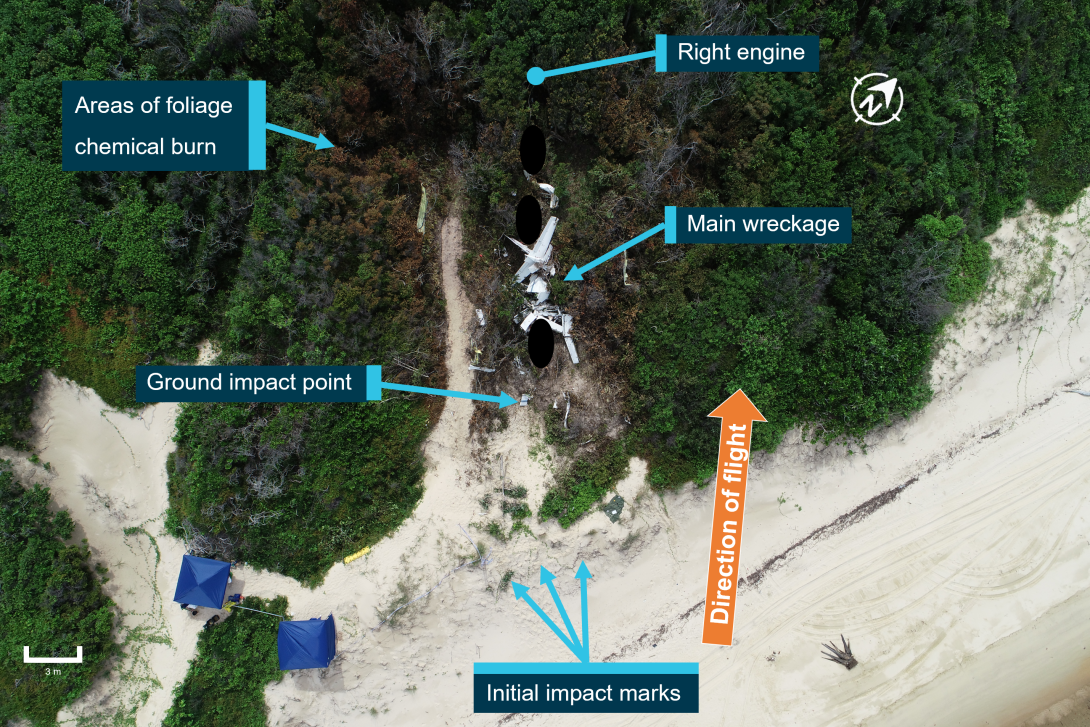
Source: ATSB
The ATSB’s on-site examination of the wreckage, damage to surrounding vegetation and ground markings indicated that at initial impact the aircraft was:
- upright and close to wings level
- at a flight path angle of about 5° nose down
- at relatively high speed.
An area of foliage around the aircraft displayed signs of chemical burn from avgas, indicating that the aircraft had a significant amount of fuel on board. There was no evidence of any structural or mechanical defects, but the examination was limited by the extensive damage.
The damage to the recovered propellor blades indicated significant rotational energy at impact consistent with both engines operating normally with substantial power.
The landing gear was extended at the time of impact. Other aircraft configuration information such as flap position, trim settings and switch selections could not be validated due to the impact damage.
The only components on the aircraft that may have recorded data were a digital fuel flow indicator/totaliser and a transponder, and the ATSB recovered these components. After consideration of the damage to these components and the potential value of any data, no further examination was undertaken.
Survivability aspects
Given the aircraft’s speed at impact (about 150 kt or 278 km/h) and the resultant impact forces (as evidenced by the nature of the wreckage), the accident was not considered survivable.
The aircraft was not fitted with a fixed emergency locator transmitter (ELT), nor was it required to be under the current regulations. A personal locator beacon (PLB) was in the pilot’s flight bag. Post-accident onsite examination noted it was within its expiry date and it passed a function test. PLBs do not have an inertial g-switch to automatically switch them on when an accident occurs, so the PLB did not activate during the accident sequence.
At 0934 and 0938, air traffic control (ATC) attempted to contact the pilot of VH-OZO after they had not cancelled or amended the SARTIME[59] of 0930. ATC also requested that the pilot of an inbound aircraft attempt to contact VH-OZO on the CTAF.
At 0939, an INCERFA was declared (which is a situation where uncertainty exists regarding the safety of an aircraft and its occupants) and soon after ATC transferred management of the situation to the Joint Rescue Coordination Centre (JRCC). At 1020, the JRCC advised ATC that it had declared a DETRESFA or distress phase (which is a situation where there is reasonable certainty that an aircraft and its occupants require immediate assistance). At 1251, the JRCC advised the ATSB that the wreckage at been located.
Organisational information
Air Connect Australia
Air Connect Australia was issued with an Air Operator’s Certificate (AOC) by the Civil Aviation Safety Authority (CASA) in March 2017 with an expiry date of 31 March 2020. It authorised the certificate holder to operate Cessna C310/340, C404, C402/421 and Raytheon Baron/Travelair twin piston-engine aeroplane types as well as single piston-engine aeroplane types with a MTOW not exceeding 5,700 kg on charter and aerial work operations.
At the time of the accident, CASA was assessing the operator’s application to renew the AOC, which was subsequently issued on 1 May 2020 with an expiry date of 30 September 2023.
From April 2017, the operator dry-leased VH-OZO from the aircraft owner, who was based in Western Australia. In this arrangement, the aircraft owner was responsible for the continuing airworthiness of the aircraft and the operator managed the operational aspects, such as fuel and flight crew.
Initially the operator’s personnel consisted of a chief pilot and the managing director, who was also the head of aircraft airworthiness and maintenance control. The chief pilot and managing director were the operator’s only line pilots. The chief pilot left in 2018, and the manager director became the chief pilot following an assessment by CASA.
In the 18 months prior to the accident, VH-OZO was the only aircraft operated and the operator employed one pilot (the pilot of the accident flight) additional to the chief pilot. As previously stated, the pilot of the accident flight conducted most of the operator’s flights.
Operator proficiency checks
To conduct IFR flights in a multi-engine aircraft, a pilot was required to complete an instrument proficiency check (IPC) every 12 months. The checks had to be conducted by a CASA-approved flight examiner. There were no additional requirements for proficiency checks for pilots conducting passenger charter operations under the IFR unless the operator had a check and training organisation as specified in Civil Aviation Regulation 217 (Training and checking organisation), which did not apply to operators such as Air Connect Australia.
CASR Part 135 (Australian air transport operations—smaller aeroplanes) was registered in December 2018 and commenced on 2 December 2021. It included a requirement for operators to conduct proficiency checks on pilots, with the requirements for such checks to be specified in the Manual of Standards (MOS). The draft Manual of Standards (MOS) for Part 135, publicly consulted in September 2018, included specific requirements for recurrent proficiency checks. For operators conducting IFR flights or flights at night, an operator proficiency check (OPCs) was required about 6 months after commencing unsupervised line operations for the operator and subsequently at intervals of 6 months. The MOS for Part 135 that came into effect in December 2021 had effectively the same requirements (with an OPC now called a ‘flight crew member proficiency check’).
Version 2 of the operator’s operations manual (February 2018) required an OPC to be conducted ‘every year’, with another section stating these needed to be completed ‘within the previous 12 months’. Version 3 of the manual (February 2020) required an OPC be conducted at the chief pilot’s discretion at periods not exceeding 24 months.[60] The manual stated that the OPC could be conducted by the chief pilot or a designated flight examiner.
The chief pilot noted that the flight examiner who regularly conducted IPCs for the operator (and was designated to conduct OPCs) would effectively conduct an OPC when they did an IPC. The change to every 24 months was done to provide more flexibility for scheduling checks.
In terms of the nature or content of the OPC, the operations manual stated that an OPC was required to be conducted
…on a flight encompassing all operations in which the pilot would normally be engaged. These flights will cover flight planning, refuelling, aircraft weight and balance, passenger briefing, forced landings and all emergency operations.
The manual also included an OPC form, which listed 23 items to be evaluated.
As previously discussed (Qualifications and experience), after the pilot of VH-OZO was cleared for line operations on 29 October 2018 (which included an OPC), the operator did not conduct any OPCs on the pilot. In addition, the chief pilot did not conduct any flights with the pilot after October 2018.
The pilot’s IPC in August 2019 was conducted with a flight examiner who was not familiar with the operator’s operations manual and therefore did not cover all aspects of an OPC, and the additional supervised training in December 2019 for night recency was done by an external provider and did not evaluate line operations.
Monitoring of pilot recency
CASR Part 61 outlined several different recency requirements. With relevance to the accident flight, these included a pilot not being able to:
- conduct a flight with passengers by day in a particular category of aircraft unless had conducted 3 take-offs and landings within the previous 90 days in that category of aircraft (CASR 61.395 (1))
- conduct a flight under the IFR unless had conducted at least 3 instrument approaches within the previous 90 days (CASR 61.870 (2))
- conduct a flight under the IFR in a particular category of aircraft unless had conducted at least 1 instrument approach in the previous 90 days in that category of aircraft (CASR 61.870 (3))
- conduct a 2D instrument approach unless had conducted at least one such approach in the previous 90 days (CASR 61.870 (4))
- conduct a flight under the IFR in a single-pilot operation unless had conducted at least one single-pilot flight under the IFR in the previous 6 months that had a duration of at least 1 hour and involved one instrument approach (CASR 61.875).
The CASA website stated:
We use recent experience requirements to maintain a pilots knowledge and skills when conducting instrument approach operations.
When conducting an approach to satisfy a recent experience, pilots should recognise the purpose of the approach is to maintain their competency to conduct such operations.
Simulating IMC, when safe to do so, will enhance the purpose of the approach. Conducting the approach provides some effective practice…
As previously noted, the pilot had been regularly logging the conduct of RNAV GNSS approaches (Qualifications and experience) as well as other types of instrument approaches. The pilot met all required recency requirements listed above.
The operator used the Aerotrack aviation management tool. The tool could be used to manage flight scheduling, fleet operations, and crew recency and rostering to meet regulatory requirements. It also created an online pilot logbook, as it tracked all flights conducted for the operator, and totalled recency requirements such as IFR flight time and instrument approaches.
The Aerotrack system correctly tracked all of the recency requirements except for CASR 61.875. The system tracked the total number of single-pilot IFR flight hours over a rolling 6-month period, calculated as a sum of all hours logged including partial hours (such as 0.5 hours).[61] However, it was not programmed to track if a single-pilot flight under the IFR (of at least 1 hour duration) was conducted.
Regulatory oversight
In June 2017, CASA conducted a level-1 surveillance event on Air Connect Australia. CASA concluded that the operator was:
an overall compliant organisation with sufficiently equipped facilities and suitable qualified personnel conducting operations in accordance with its legislative authorisations and responsibilities.
The audit identified 5 non-compliance notices, including 3 relating to operations manual content regarding transponders, daily inspections and oil consumption records, and 2 relating to incomplete staff induction records for one pilot and the emergency proficiency checks for one pilot carried out by a person who was not the chief pilot. Other findings included 2 observations relating to job descriptions and one aircraft survey report finding. These findings were addressed by the operator.
In June 2018, CASA conducted a level-2 airworthiness and maintenance system surveillance activity relating to VH-OZO, prior to a flying operations inspector flying on the aircraft for the purpose of a chief pilot assessment. The surveillance report found there were no anomalies identified in either the technical documentation assessment or the physical inspection of the aircraft and the chief pilot assessment was then conducted.
In February 2020, CASA conducted a desk-top surveillance activity to determine if the AOC could be subsequently re-issued. The surveillance considered the organisation’s surveillance and compliance history, the management structure and operational oversight effectiveness. The review did not indicate any matter that would preclude a subsequent issue of the organisation’s AOC.
In May 2020, CASA conducted a post-accident regulatory and safety review. This review concluded that VH-OZO was correctly registered, certified for flight, maintained by qualified people, flown by a qualified person to a qualified aerodrome using a correctly validated approach. The review stated that past and current surveillance events had not detailed safety concerns with the operation of VH-OZO.
Operational information
Pre-flight planning and in-flight monitoring
Civil Aviation Regulation 239 (Planning of flight by pilot in command) stated:
Before beginning a flight, the pilot in command shall study all available information appropriate to the intended operation, and, in the cases of flights away from the vicinity of an aerodrome and all I.F.R. flights, shall make a careful study of:
a. current weather reports and forecasts for the route to be followed and at aerodromes to be used;
b. the airways facilities available on the route to be followed and the condition of those facilities;
c. the condition of aerodromes to be used and their suitability for the aircraft to be used; and
d. the air traffic control rules and procedure appertaining to the particular flight;
and the pilot shall plan the flight in relation to the information obtained.
AIP ENR 1.10 (Flight Planning) also stated these requirements. In addition, it stated a pilot was required to review NOTAMs[62] applicable to the flight.
At 1326 on the day before the accident flight, the pilot accessed a location briefing for Lockhart River from the National Aeronautical Information Processing System (NAIPS) via an electronic flight bag (EFB) application. This type of briefing typically displayed current forecasts, reports, and ‘notice to airmen’ (NOTAM) applicable to the nominated location.
Later that day, at 1830, the pilot requested grid point wind and temperature charts (GPWT) and a specific pre-flight information bulletin (SPFIB) from NAIPS via flight planning software. The SPFIB request was for Cairns to Lockhart River and return with the estimated time of departure nominated as 1930 the same day. This bulletin was valid until 1830 on the day of the accident.
A printout of the SPFIB found at the accident site showed aerodrome forecast (TAF) and weather reports (METAR) for Cairns. The weather for the next day (day of accident flight) at Cairns Airport was expected to be a visibility of 10 km or greater and showers of light rain with scattered cloud at 1,800 ft in the morning lifting to 2,500 ft. In addition, the forecast imposed a TEMPO for the next day to specify periods of visibility reduced to 2,000 m with showers of moderate rain and broken cloud at 1,000 ft.
On the printout of the SPFIB, a METAR for Lockhart River for 1800 (10 March 2020) showed light winds, visibility 10 km or greater and nil cloud detected. Since 0900 that morning, recorded rainfall was 1.8 mm.
No TAF was provided on the SPFIB for Lockhart River as the time of the request was outside the issue and validity period. There were no predicted outages of global positioning system/global navigation satellite system (GPS/GNSS) capability for Cairns or Lockhart River. NOTAM information included a change to Lockhart River runway distance and gradient data and no other notices with significance for the planned flight.
After the SPFIB was received, at 1942, the pilot submitted a flight notification for the planned departure from Cairns at 0730 the next morning to Lockhart River followed by a departure at 1430 for the return sector. Both sectors were planned under IFR with nominated capability for instrument approaches using GPS/GNSS equipment.
After the pilot requested the SPFIB and submitted the flight notification on the evening before the accident flight, there was no record of further requests for meteorological information from NAIPS. Such information was also available from the Bureau of Meteorology website and other sources without any user registration requirements. The ATSB was advised that the pilot was aware of the current weather forecasts for Lockhart River and Cairns on the morning before the flight.
A damaged and partly illegible copy of the pilot’s flight plan/log was found at the accident site. This was a printout from flight planning software showing key navigational data and pilot notes on progress of the flight. There was no indication of any operational abnormalities.
Fuel calculations
On the morning of the flight, the pilot refuelled the aircraft with 650 L of avgas. A tabulated fuel plan showed 1,040 L on board at engine start at Cairns and expected fuel consumption of 285 L for the planned 94-minute flight to Lockhart River. The pilot had included provision for 45 minutes fixed reserve (124 L), 40 L variable reserve and 60 minutes holding (110 L) if required (consistent with the forecast TEMPO conditions in the TAF, see Aerodrome forecasts). If the variable reserve and holding allowance was consumed on the outbound sector (in addition to the calculated flight fuel), the remaining 605 L was sufficient to return to Cairns with allowance for 60-minutes holding on arrival.
In summary, the aircraft had sufficient fuel to conduct the flight from Cairns to Lockhart River and return, with additional fuel for holding on both sectors if required. The pilot was probably not intending to refuel at Lockhart River, although avgas was available if required.
Weight and balance
The pilot completed the operator’s passenger/cargo manifest form and calculated the aircraft’s weight and balance with reference to individual passenger weights and baggage. The take-off weight was recorded as 3,678 kg and nominal landing weight as 3,366 kg (7,421 lb). The aircraft’s maximum take-off weight was 3,810 kg and maximum landing weight was 3,674 kg. The graphical trimsheet showed the centre of gravity was within limits throughout the flight.
A copy of the operator’s in-flight monitoring form was found at the accident site. When the pilot completed the form in cruise at 10,000 ft, all of the recorded engine parameters for each engine were comparatively similar with no indication of any aircraft-related problems.
Communications during approaches
The AIP (ENR 1.1, 10.1) recommended radio calls at an uncontrolled aerodrome. These included:
- the pilot being inbound to an aerodrome (10 NM or earlier, commensurate with aeroplane performance and pilot workload, with an estimated time of arrival)
- during an instrument approach:
- departing the FAF or established on the final approach segment (including details of position and intentions)
- terminating the approach or commencing the missed approach (including details of position and intentions).
The Air Connect Australia operations manual stated the following for communications at non-towered aerodromes:
When operating within the vicinity of a non-controlled aerodrome, a known training area or authorised low flying area, Company pilots are to broadcast their intentions, listening out and communicate with any possible conflicting aircraft in accordance with the requirements of the AIP.
Additionally, for straight-in approaches at non-towered aerodromes, the manual stated:
- Monitor / broadcast on CTAF shall be made by 10 NM and include aircraft type, position, callsign and include the intention to make a straight-in approach.
- Monitor / broadcast at 3 NM that the aircraft is established on the final approach.
During both of the approaches at Lockhart River, the pilot of VH-OZO made broadcasts when at 10 NM (that is, at about the IF). During the first approach, the pilot also made a broadcast at 5 NM (at the FAF) and during the missed approach. There was no call recorded when the aircraft was at 5 NM on the second approach.
Electronic flight bag and approach charts
During the flight, the pilot was using an iPad with an electronic flight bag (EFB) application (OzRunways) and was carrying a second iPad as a backup. The operator’s operations manual (section 2A1.3.2.2) stipulated that all EFB devices permitted for use were class 1 (portable electronic device) and functionality level 1, which meant that it could be one or more of the following:
- held in the hand
- mounted on an approved mount
- attached to a stand-alone kneeboard secured to a flight crew member
- connected to the aircraft power for battery re-charging
- connected to an installed antenna intended for use with the EFB for situational awareness but not navigation.
Furthermore, unless secured in accordance with b or c above, the EFB was required to be stowed during take-off, landing, instrument approach and when flying less than 1,000 ft above terrain. It was also only to be used:
…as a source of navigational data e.g. Departure and Approach plates and airport information.
The operator did not have an approved mount for the iPad. Accordingly, the operator required paper approach charts to be used when conducting an instrument approach. The chief pilot stated that the pilot subscribed to the departure and arrival procedure charts published by Airservices Australia. There was a clamp on the control column where a paper approach chart could be placed (Figure 8). There were no paper approach charts identified at the accident site, although the flight plan/log was found. Due to the disruption of the wreckage, it could not be determined whether the pilot carried the paper charts on board.
A friend of the pilot stated that they had provided the pilot with a mounting device that could be used to mount an iPad on a control column. The pilot’s iPad EFB was on[63] during the approach, however there were differing reports about how the pilot used the iPad during previous flights and whether it was mounted on the control column, placed on their knee or placed on the vacant seat next to the pilot. Due to disruption of the aircraft in the accident, only the second (backup) iPad was found at the accident site.
Flight profiles
The operator’s operations manual included flight profiles to be used for various situations for the Cessna 404, and stated that the profiles were required to be used for all operations. The prescribed flight profile for an RNAV GNSS approach (and other straight-in instrument approaches) is shown in Figure 19.
The indicated airspeed was required to be below 180 kt at the IAF and at 130 kt (+ or - 5 kt) at the start of the descent after passing the IAF (with the landing gear down and approach flap selected). The airspeed was also required to be Vref to Vref +10 kt at the FAF. As noted in Configurations and speeds, the Vref at maximum landing weight (8,100 lb) for the Cessna 404 was 91 kt and at weights 7,500 lb and below was 88 kt. In effect, the operator’s flight profile requirement to be at Vref to Vref + 10 kt at the FAF equated to an indicated airspeed of about 90–100 kt.
Figure 19: Flight profile for Cessna 404 RNAV GNSS approach
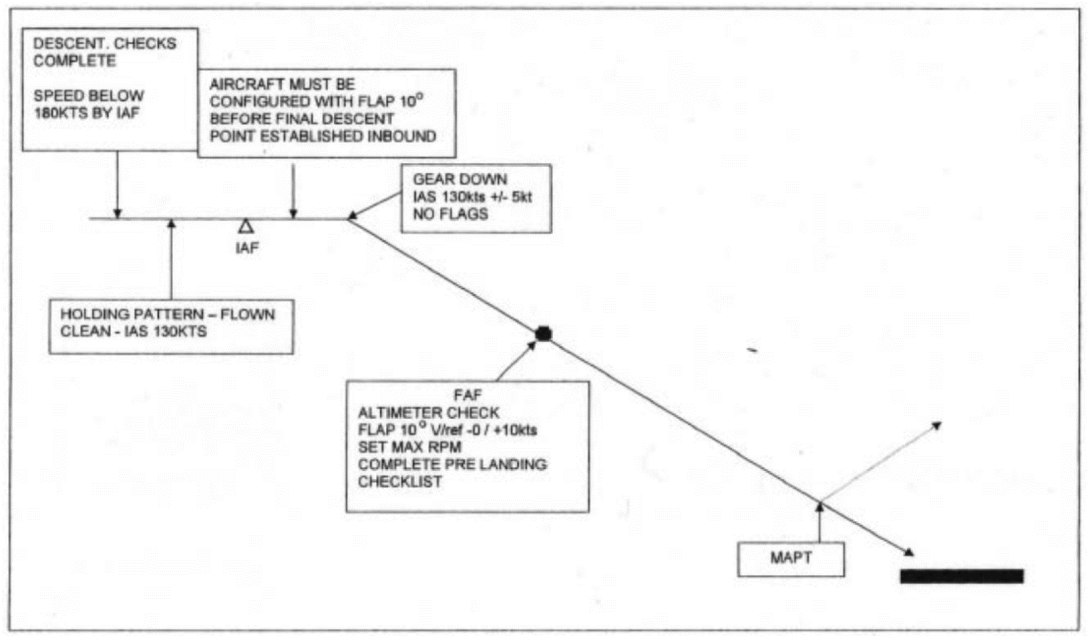
In another section titled ‘Final approach & threshold speeds’, the operations manual stated:
- The [pilot] shall conduct the final approach in accordance with the stabilised approach criteria… [see next section]
- CAT B: Speeds 91 – 120 kts. Initial / Intermediate 120 – 180 kts. Final app 85 – 130 kts. Visual circling 135 kts. Max speed for Missed Approach 150 kts.
These were the ‘handling speeds’ referred to the AIP for instrument approaches for a category B aircraft, such as the Cessna 404 (see Handling speeds). In effect, these speeds meant that the maximum allowed indicated airspeed after passing the IAF for the Cessna 404 was 180 kt and the maximum airspeed after passing the FAF was 130 kt.
The chief pilot recalled that the flight profiles were included in the operations manual by the previous chief pilot, who had based them on profiles used by another operator. The chief pilot stated that they advised the pilot of the accident flight to be at about 5,000 ft at the start of an approach (at the IAF) and then use a continuous descent to the runway from the IAF, and they demonstrated this to the pilot during their supervised flying in October 2018.
The chief pilot recalled that, when they flew the aircraft, they would select approach flap and landing gear early in the descent and be stabilised at a speed of 120 kt and a descent rate of 600 ft/min[64] well before the FAF. The chief pilot stated that the speed at the FAF should be about Vref + 20 kt (which equates to about 110 kt) but that the 100 kt specified in the flight profile would be acceptable. They stated that 130 kt at the FAF was too fast.
The previous chief pilot also recalled that the operator’s flight profile was most likely obtained from another operator. They noted their recollection of speeds was limited given the time since they flew the aircraft, but they recalled that they would be at least at Vref + 10 kt (that is, 100 kt) but not faster than 120 kt at the FAF, then slowing the aircraft down by 1,000 ft.
The flight examiner regularly used by the operator also worked with another operator of Cessna 404 aircraft (which was a different operator to that referred to by the previous chief pilot). The examiner stated that at the IF they would normally be at 130 kt with gear down and approach flap selected. The normal speed they used at the FAF was 110 kt (Vref + 20 kt), and 100 kt would be a little slow at that point on the approach. The examiner advised that these speeds were also used by the other Cessna 404 operator.
In summary, the ATSB concluded that the operator’s published flight profile speed of 90–100 kt at the FAF was probably not the operator’s preferred speed during flight operations. Rather, it appeared that the preferred speed at the FAF was probably about 110 kt. However, the extent to which this was clearly communicated to the pilot of the accident flight could not be determined.
Stabilised approach criteria
Guidance regarding stabilised approach criteria
A stabilised approach is one in which all criteria specified in the operations manual are met, at or before the applicable height or reference point. The Flight Safety Foundation (FSF) has for many years recommended that operators have stabilised approach criteria. Detailed guidance was provided by the FSF in its approach and landing accident reduction (ALAR) briefing note 7.1 (Stabilized approach, 2000a). The recommended criteria were summarised in a list, as reproduced in Figure 20.
Figure 20: FSF recommended elements of a stabilised approach
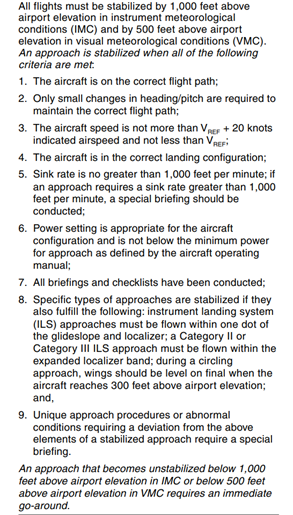
Source: ALAR briefing note 7.1 (Flight Safety Foundation 2000a)
The FSF briefing note also stated:
The flight crew must “stay ahead of the aircraft” throughout the flight. This includes achieving desired flight parameters … during the descent, approach and landing. Any indication that a desired flight parameter will not be achieved should prompt immediate corrective action or the decision to go around.
The minimum stabilization height constitutes an approach gate on the final approach; a go-around must be initiated if:
- The required configuration and airspeed are not established, or the flight path is not stabilized when reaching the minimum stabilization height;
- The aircraft becomes unstabilized below the minimum stabilization height.
ICAO Annex 6 Part I applied to international commercial air transport operations in aeroplanes. Since 1998, it included a standard stating an operator’s operations manual had to include stabilised approach procedures.
ICAO document 8168 (Procedures for Air Navigation Services, Aircraft Operations, known as PANS-OPS) provided recommendations on procedures for flight crew. Since 2001, PANS-OPS included content on stabilised approaches and stabilised approach criteria. It stated:
Studies have shown that the risk of controlled flight into terrain (CFIT) is high on non-precision approaches. While the procedures themselves are not inherently unsafe, the use of the traditional step down descent technique for flying non-precision approaches is prone to error, and is therefore discouraged. Operators should reduce this risk by emphasizing training and standardization in vertical path control on non-precision approach procedures…
Operators should use the CDFA (continuous descent final approach) technique whenever possible as it adds to the safety of the approach operation by reducing pilot workload and by lessening the possibility of error in flying the approach…
This technique requires a continuous descent, flown either with vertical navigation (VNAV) guidance calculated by on-board equipment or based on manual calculation of the required rate of descent, without level-offs. The rate of descent is selected and adjusted to achieve a continuous descent to a point approximately 15 m (50 ft) above the landing runway threshold or the point where the flare manoeuvre should begin for the type of aircraft flown...
The guidance document also stated:
The primary safety consideration in the development of the stabilized approach procedure shall be maintenance of the intended flight path as depicted in the published approach procedure, without excessive manoeuvring.
PANS-OPS stated the types of information that should be included in stabilised approach criteria (such as speeds, minimum power settings, attitudes, crossing altitude deviation tolerances, aircraft configuration, maximum sink rate and competition of checklists and briefings). In addition, the document stated that an operator’s procedures should include, as a minimum:
a) that in instrument meteorological conditions (IMC), all flights shall be stabilized by no lower than 300 m (1 000 ft) height above threshold; and
b) that all flights of any nature shall be stabilized by no lower than 150 m (500 ft) height above threshold.
Although the FSF and ICAO guidance may be considered most applicable to larger air transport aircraft with multi-crew operations and turbine engines, similar guidance (with the same applicable heights of 1,000 ft for operations in IMC and 500 ft for operations in VMC) has also been widely recommended for operations in smaller aircraft (Appendix D – Guidance to industry regarding stabilised approaches). This guidance has emphasised the benefits of using a CDFA technique with stabilised approach criteria in terms of reducing workload and increasing the time to monitor, detect and react to problems.
In Australia, since 2014, the Civil Aviation Advisory Publication (CAAP) 215-1 (Guide to the preparation of operations manuals) stated that an operator’s manual should include stabilised approach criteria in its section on approach and landing procedures. Guidance regarding applicable heights or reference points for such criteria were not specified in the CAAP or other CASA guidance documents.[65]
CASA advised the ATSB that specific Australian guidance was not provided prior to 2021 since there was no direct legislative requirement for operators to use such criteria, and it was considered that there was readily available and significant global guidance on this topic available from a wide range of authoritative sources.
Operator’s stabilised approach criteria
The Air Connect Australia operations manual stated:
Stabilised Approach Criteria are as follows:
(a) All flights, other than training flights, must be stabilised by 300 feet above aerodrome elevation in both IMC and VMC.
(b) An approach is stabilised when the following criteria are met:
- The aircraft is on the correct flight path;
- Only small changes in heading and pitch are required to maintain the correct flight path;
- The aircraft is not more than Vref + 20 kts and not less than Vref indicated airspeed
- The aircraft is in the correct landing configuration;
- Sink rate is no greater than 1000 fpm [ft/min];[66] if an approach requires a sink rate greater than 1000 fpm, a special briefing should be conducted;
- Power setting is appropriate for the aircraft configuration and is not below the minimum power for approach as defined by the aircraft operating manual;
- All briefings and checklists have been conducted;
- Instrument approaches are stabilised by the final approach fix, if they also fulfil the tracking requirements – established within ‘half scale deflection’ for the ILS, VOR and GNSS, within + or – 5 degrees for the NBD; during a circling approach, wings should be level on final by 300 feet above aerodrome elevation;
- Unique approach procedures or abnormal conditions requiring a deviation from the above elements of a stabilized approach require a special briefing.
Missed Approach Procedure is as follows:
(a) Other than on training flights, an approach that is not stable below 300 feet aerodrome elevation in both IMC and VMC requires an immediate GO-AROUND. The procedure for a go around / missed approach is as follows:
- Should be flown as per the approach chart missed approach procedure, or as advised by ATC;
- The aircraft handling technique will be as per the relevant AFM for the appropriate aircraft…
The manual further stated:
If an approach does not meet the criteria for a stabilised approach…, the Pilot-in-Command shall conduct a missed approach.
To meet these stabilised approach criteria, the maximum indicated airspeed in a Cessna 404 needed to be Vref + 20 kt or about 110 kt at 300 ft above aerodrome elevation.
The chief pilot advised the ATSB that the published applicable height of 300 ft was too low to be effective, as it was normally below the MDA. They believed approaches should be stabilised much earlier, ideally at height of about 1,000 ft or even earlier. The chief pilot also stated that all configuration changes should be done early in the approach, with only the selection of landing flap to be completed late in the approach at the pilot’s discretion. The flight examiner used by the operator noted that stabilised approach criteria for most piston twin-engine and single-engine aeroplanes needed to acknowledge that the last stage of flap (or landing flap) should generally not be selected until about 300 ft due to aircraft performance considerations.
The chief pilot also recalled that they had discussed the importance of being stabilised early in the approach to the pilot of the accident flight on multiple occasions, and they had stated the importance of conducting constant angle descents down to the MDA at 600 ft/min. The chief pilot also noted that they had emphasised to the pilot the importance of gradual reductions in power and therefore speed during approaches in order to minimise undesirable cylinder head temperatures, and use slow descent rates for passenger comfort.
Additional information
During its investigation into the 2005 CFIT accident of a Metro aircraft at Lockhart River involving an operator that conducted passenger transport operations in Metro turboprop aircraft, the ATSB identified that that operator did not have stabilised approach criteria.[67] Of 5 other operators conducting operations in Metro aircraft, all had stabilised approach criteria, with 1 having an applicable height of 200 ft, 2 having an applicable height of 300 ft and 2 having an applicable height of 1,000 ft.
The ATSB also identified that an operator conducting passenger transport operations in a DHC-8 aircraft in 2012 had stabilised approach criteria based on an applicable height of 300 ft.[68] In addition, during another investigation commenced in 2021, the ATSB recently identified another operator of turboprop aircraft conducting passenger transport operations (charter) that had stabilised approach criteria with an applicable height of 300 ft. The ATSB has also identified that major airlines and some operators of single-pilot IFR operations in Australia have criteria based on 1,000 ft above ground level in IMC.
Prior missed approach during an RNAV GNSS approach (22 January 2020)
A review of the pilot’s logbook identified one other flight since the pilot joined the operator that involved 2 instrument approaches (and therefore a missed approach following an instrument approach). This occurred on a flight from Weipa to Aurukun, Queensland, on 22 January 2020, after which the pilot logged 2 RNAV GNSS approaches. Recorded data confirmed that the pilot conducted 2 approaches to runway 34 at Aurukun.
Analysis of the weather conditions and interviews with the passengers identified that there was a storm in the vicinity at the time, and interviews and recorded flight data indicated that the pilot was trying to avoid the weather. The passengers recalled observing the pilot using the onboard weather radar as well as an iPad with the weather on it to track the movement of the storm.
Passenger recollections regarding the weather during the first approach were varied; some recalled that they could not see the runway at times whereas others recalled the runway was still visible though restricted. The ground appeared to be visible most of the time. Images taken by a passenger during the first approach confirmed that there was significant cloud in the area though the ground could be seen to the left of the aircraft.
Review of the recorded flight data indicated that, on the first approach, the aircraft commenced descent on the recommended descent profile from about 1,600 ft, slightly below the initial approach altitude of 1,800 ft. The aircraft passed the FAF at an indicated airspeed of about 140 kt, and it was about 200–300 ft below the recommended descent profile when it passed the MDA at an indicated airspeed of about 145 kt. The aircraft descended to a recorded altitude of about 200–300 ft (at about 140 kt) before starting to climb.
The missed approach did not conform to the published missed approach procedure, with the aircraft flying to the right of the MAPt rather than maintaining the runway heading. However, the conditions may have been visual at this time. After the missed approach, the pilot circled for some time, remaining in VMC and waiting for the storm to pass, before conducting a second approach (about 31 minutes after the first approach). Images taken by a passenger during the second approach confirmed that the weather conditions were significantly better than on the first approach.
The exact reasons for the missed approach could not be determined (that is, whether it was due to reduced visibility of the runway or also due to the aircraft’s speed not meeting the operator’s stabilised approach criteria at 300 ft above aerodrome elevation).
Further details of these 2 approaches are provided in Appendix E – Aurukun incident flight – 22 January 2020.
Review of the pilot’s recent RNAV GNSS approaches
General aspects
The ATSB reviewed the available recorded data for the pilot’s flights in the previous 6 months for which the pilot had logged an RNAV GNSS approach (that is, 21 approaches). Recorded data was available for 16 of these approaches. Of these 16 approaches:
- 10 involved straight-in approaches to the runway
- 5 were flown to past the FAF and then a circling approach was flown from above the specified circling minima to the opposite (reciprocal) runway
- 1 was not an RNAV GNSS approach as it did not fly near the designated waypoints (and it was therefore not considered for further analysis).
Based on a review of available weather information, all 21 approaches were very likely conducted in VMC except for the first approach conducted at Aurukun on 22 January 2020 (which was potentially in IMC for a brief period). For those approaches conducted in VMC, the pilot was not specifically required to conduct an RNAV GNSS approach, except for the purpose of meeting recency requirements.
The published approach charts for all the approaches had 5 NM segments between the IAF and IF, IF and FAF, and FAF and MAPt. Most of the approaches were conducted at locations where the recommended descent profile commenced at about the FAF, except for one approach to runway 11 at Cooktown (where the recommended descent profile commenced at the IAF).
Vertical profiles
For most of the 15 RNAV GNSS approaches with recorded data available, the data showed the pilot typically descended to about 5,000–5,300 ft and then levelled out for a short period prior to the IAF. When passing the IAF, the pilot commenced a continuous descent to join the approach’s recommended descent profile on about a 3° approach.
The only exceptions to this method were the 2 approaches conducted at Aurukun on 22 January 2020 (when the pilot commenced descent on the approaches from close to the published initial approach altitude of 1,800 ft). As noted in Recorded flight data, the second approach at Lockhart River during the accident flight was also commenced at a lower altitude (that is, the published initial approach altitude of 3,500 ft) when the aircraft was between the IAF and the IF).
In general, the approaches were conducted close to the recommended descent profile. The only exception was the first approach at Aurukun on 22 January 2020, which reached the MDA about 200–300 ft below the recommended profile.
Lateral positions
The pilot generally entered each approach via the nearest of the 3 IAFs. In most cases, the aircraft passed the IAF from close to the middle of the capture region for that waypoint, or at least on a track that was less than 45° difference to the initial approach track. On one approach (at Cooktown), they entered from a similar position as the second approach at Lockhart River, although from about 65° right of the extended initial approach track to the IAF labelled ‘A’ (as opposed to about 100° at Lockhart River).
For all the straight-in runway approaches (except the second approach at Lockhart River), the aircraft remained close to the intermediate approach track and final approach track. In most cases, the aircraft also remained close to the initial approach track. The only exceptions were:
- the Cooktown approach (when the aircraft flew over the IAF then, while descending, deviated left of the initial approach track by 0.9 NM and remained left until close to the IF)
- the first approach at Aurukun on 22 January 2020 (when the pilot commenced the approach from slightly more than 1 NM west of the IAF and flew direct to the IF from that position, while remaining close to the initial approach altitude).
Indicated airspeeds
The ATSB reviewed the indicated airspeeds during the pilot’s 8 previous straight-in RNAV GNSS approaches to evaluate their consistency with the operator’s speed requirements and preferences, and these were compared with the 2 approaches at Lockhart River on the day of the accident (Figure 17). The 8 other approaches included the 2 approaches conducted at Aurukun on 22 January 2020 (Figure 25), and 6 other approaches that the pilot conducted from December 2019 to February 2020 and logged as RNAV GNSS approaches.
For the 10 approaches, the ATSB estimated the indicated airspeeds based on the recorded groundspeed, forecast and analysis wind charts and other relevant information. The actual wind speeds above the aerodrome on each occasion were not known, and as a result there could have been some differences between the estimated indicated speeds and the actual indicated airspeeds.
The results for key points on the approaches are shown in Table 4. In general terms:
- In all 10 cases, the approaches did not exceed (and were well below) the maximum AIP handling speed of 180 kt in the initial and intermediate approach segments.
- In all 10 cases, the approaches exceeded the operator’s preferred speed of 110 kt at the FAF. Most of the approaches were about 130–140 kt at the FAF, and 7 of the approaches appeared to exceed the maximum AIP handling speed of 130 kt at or after passing the FAF by 10 kt or more.
- In most cases, the approaches appeared to be close to the operator’s maximum stabilised approach speed of 110 kt at or approaching 300 ft. However, in 3 cases there was a significant exceedance. These included the first approach at Aurukun on 22 January 2020 (which was followed by a missed approach), and the 2 approaches at Lockhart River (with the first followed by a missed approach).
Table 4: Summary of estimated indicated airspeeds during the pilot’s recent RNAV GNSS approaches (in kt)[69]
IF | FAF | FAF | 1,000 ft | 300 ft | |
Operator requirement or preference | (180 max) | 110 preferred | (130 max) | (130 max) | 110 max |
Mornington Island, 2 Dec 2020 (VMC) | 145 | 140 | 140 | 140 | 120 |
Normanton, 9 Dec 2020 (VMC) | 145 | 145 | 145 | 145 | 125 |
Cooktown, 4 Jan 2020 (VMC) | 140 | 140 | 140 | 140 | 115 |
Aurukun, 22 Jan 2020 (potential IMC, missed approach) | 140 | 140 | 140 | 140 | 140 |
Aurukun, 22 Jan 2020 (VMC, second approach) | 135 | 135 | 135 | 125 | 105 |
Aurukun, 3 Feb 2020 (VMC) | 150 | 145 | 145 | 145 | 115 |
Kowanyama, 13 Feb 2020 (VMC) | 140 | 120 | 120 | 120 | 115 |
Pormpuraaw, 18 Feb 2020 (VMC) | 150 | 130 | 130 | 120 | 115 |
Lockhart River, 11 Mar 2020 (IMC, missed approach) | 135 | 130 | 130 | 140 | 1401 |
Lockhart River, 11Mar 2020 (IMC, second approach, accident) | 135 | 135 | 135 | 140 | 150 |
The requirement at 300 ft above aerodrome elevation is from the operator’s stabilised approach criteria. The maximum requirements for the IF, FAF and 1,000 ft above aerodrome elevation are from the AIP handling speeds for a category B aircraft (also referred to in the operations manual). The operator preferred speed at the FAF is based on interviews. All speeds rounded to nearest 5 kt. Green shading indicates the speed was consistent with the requirement or preference (within 5 kt), orange shading indicates a small exceedance (within 10 kt), and red shading indicates a significant exceedance (15 kt or more).
1Descent to just above 300 ft above aerodrome elevation (or just below that height) on this approach occurred after passing the MAPt.
In summary, the pilot was not conducting RNAV instrument approaches in the Cessna 404 in accordance with the operator’s preferred speed of 110 kt at the FAF. In some cases, the pilot appeared to be complying or was close to the AIP handling speed requirement to be at a maximum speed of 130 kt in the final approach segment, but in some cases they exceeded this requirement. The chief pilot advised that they were unaware that the pilot was conducting RNAV approaches with a speed that was significantly above 110 kt at the FAF.
Controlled flight into terrain
CFIT accident data
The ATSB research report CFIT: Australia in context 1996 to 2005 (ATSB, 2007), defined a controlled flight into terrain (CFIT) as an in-flight collision with terrain, water, or obstacles, in which:
- the aircraft is under the control of the pilot(s)
- there is no defect or unserviceability that would prevent normal operation of the aircraft
- the pilot(s) had little or no awareness of the impending collision.
In the 10-year reporting period for that research (1996–2005), there were 27 CFIT occurrences in Australia. Of these 27 occurrences:
- 25 were accidents, including 15 fatal accidents, resulting in a total of 47 fatalities.
- 19 involved aeroplanes (including 18 accidents and 12 fatal accidents) and 8 involved helicopters (including 7 accidents and 3 fatal accidents)
- 17 (63%) occurred during the approach phase, with 8 during a visual approach and 9 during an instrument approach
- 1 occurred during a low-capacity regular public transport flight (RPT), 8 during charter flights, 4 during aerial work flights and 14 during private/business flights.
- only one aircraft of the 27 occurrences was fitted with a TAWS – the Fairchild SA227-DC (Metro) aircraft involved in the low-capacity RPT CFIT accident at Lockhart River in May 2005.[70] This GPWS was not a predictive or enhanced TAWS, which became required for turbine aircraft like the Metro by the end of June 2005.
Of the 9 CFIT occurrences that occurred during an instrument approach:[71]
- all 9 were accidents, including 7 fatal accidents, resulting in a total of 31 fatalities
- all 9 involved aeroplanes
- 4 occurred during an RNAV GNSS approach, 2 during a GPS arrival and 3 during an NDB approach.
- 1 occurred during a low-capacity RPT flight, 3 during charter flights, 2 during aerial work flights and 3 during private/business flights.
Further details of some of these accidents are provided in Appendix F – Related occurrences.
A review of the ATSB database for the 15-year period 2006–2020 identified only 1 CFIT involving an aeroplane on an instrument approach (that is, VH-OZO at Lockhart River, resulting in 5 fatalities). There were also 5 other CFITs involving aeroplanes on visual approaches, which resulted in 2 accidents, including 1 fatal accident with 4 fatalities.
The International Air Transport Association (IATA) (2018) published a report on worldwide CFIT accidents from 2008 to 2017 involving aircraft with a MTOW of at least 5,700 kg (12,540 lb). During that period, there were 47 CFIT accidents, which accounted for 6% of total accidents. Most (42 or 89%) of the CFIT accidents involved fatalities, and CFIT was the second highest fatal accident category (after loss of control in-flight), accounting for 28% of all fatal accidents.
Of the 47 CFIT accidents, 24 (51%) occurred during the approach phase of flight, 7 (15%) during landing, and 4 (9%) during a go-around. Turbo-prop aircraft had a much higher CFIT rate (per million flights) than jet aircraft. Older generation aircraft were also involved in more CFIT accidents.
The IATA report found that the rate of CFIT accidents was significantly lower in the last 5 years (2013–17) compared to the first 5 years (2008–12). The report stated:
It is generally accepted that the reduction in CFIT accidents can be traced back to the introduction of Ground Proximity Warning System (GPWS), and Terrain Awareness Warning System (TAWS). Other improvements, may have also contributed directly or indirectly to the reduction of the likelihood of CFIT accidents, including aircraft design, replacing non-precision with precision approach procedures, pilot training, improved flight standards, Continuous Descent Final Approach (CDFA) technique, approach lightning, visual approach guidance and procedures, ground-based Minimum Safe Altitude Warning (MSAW) system, visual and instrument approach guidance and procedures.
In addition, the IATA report noted common contributing factors to CFIT accidents, including poor visibility or IMC (49%) and lack of visual reference (33%). Unstable approaches were cited in 10% of accidents. The report stated:
Unstable approaches increase the possibility of diverting a flight crew’s attention away from the approach procedure to regain better control of the airplane. Stabilized approach policies broadly concur in stating that a safe approach requires the flight path angle, configuration, and airspeed to be stabilized. Once one or more of these parameters are violated, the approach becomes unstable and the margin for a safe landing is decreased to a level requiring flight crew action; a go-around should be initiated.
Since the CDFA techniques contribute to a stabilized approach, the industry should also as soon as, and wherever, possible to develop procedures and train pilots to fly a stabilized CDFA…
The report also stated:
It is evident that most of the CFIT accidents result from a pilot’s breakdown in situational awareness (SA) instead of aircraft malfunction or a fire. In other words, these accidents are, for the most part, entirely preventable by the pilot. SA refers to the accurate perception by flight crew of the factors and conditions currently affecting the safe operation of the aircraft, and their vertical and/or horizontal position awareness in relation to the ground, water, or obstacles. The data shows that 49 percent of CFIT accidents had vertical, lateral or speed deviations as a contributing factor to CFIT accidents. One method to provide pilots with a greater level of safety through enhanced situational awareness and, more reliable warnings of possible terrain conflicts such as EGPWS that is equipped with accurate navigation systems like global positioning system (GPS) for both navigation and terrain surveillance.
Research conducted for the Netherlands Directorate-General of Civil Aviation, under the auspices of the Flight Safety Foundation, identified a fivefold increase in accident risk in commercial aircraft flying a non-precision approach compared with a precision approach (Enders and others, 1996).
Other research conducted into the human factors associated with CFIT accidents between 2007 and 2017 found that 24 out of 50 accidents reviewed (48%) occurred during the approach phase of flight (Kelly and Efthymiou, 2019). This research also found that ‘Breakdown of the Visual Scan’ occurred in 84% of accidents and that, in 42 of the analysed accident reports, a critical parameter such as altitude was ignored, and an unsafe situation occurred through ‘distraction’, ‘complacency’ or ‘lack of skill’.
Efforts to reduce CFIT accidents
In 1995 an international CFIT task force, whose major goal was to prevent CFIT accidents, completed its work after creating several unique products for the Flight Safety Foundation (FSF) including a CFIT Education and Training Aid. The training aid described GPWS (now known as TAWS) as ‘one of the major weapons in the growing arsenal of CFIT prevention methods.’ It further recommended every aircraft be fitted with such a system, as a GPWS warning ‘is normally the flight crew’s last opportunity to avoid CFIT’.
The training aid recommended standard operating procedures and/or guidance from the aircraft manufacturer that specified flight crew actions in the event of a terrain warning. In the absence of these, the aid provided a standard escape manoeuvre for flight crew to follow in the event of a terrain warning.
The CFIT task force identified 2 ‘basic causes’ of CFIT accidents – a lack of flight crew’s vertical position awareness and their lack of horizontal position awareness in relation to the ground, water, or obstacles. More than two-thirds of all CFIT accidents were the result of altitude error or lack of vertical situational awareness. To mitigate against this, the training aid emphasised the importance of flight crew training and discipline.
CFIT mitigation strategies
In 1994, the international CFIT task force also designed a CFIT checklist for the FSF, aimed at reducing CFIT accidents (Appendix G – Flight Safety Foundation CFIT Checklist). The checklist had 3 parts:
- Part I enabled a calculation of the CFIT risk factors for the planned destinations (including air traffic control capabilities and the types of approaches available) and risk multipliers (such as the type of operation and weather conditions). Some of the risk factors relevant to this accident included no ATC at the airport and a non-precision approach type. Risk multipliers included passenger-carrying charter operation, IMC, and a single-pilot flight crew.
- Part II of the checklist listed CFIT risk-reduction factors in 4 areas: company culture, flight standards, hazard awareness and training, and aircraft equipment.
- Part III calculated the CFIT risk score by adding the destination CFIT risk factors (which are negative values) multiplied by the calculated risk multiplier to the risk-reduction factors.
The ATSB included copies of the FSF checklist in several of its accident reports, and also referred to the checklist in the research report CFIT: Australia in context 1996 to 2005 (ATSB, 2007).
In addition to the checklist, the FSF developed a CFIT Education and Training Aid in the 1990s, which included an example CFIT training program (albeit focussed on larger aircraft operations). It also developed a video training aid (for regional and business aircraft operators) and the ALAR Tool Kit, which included a number of briefing notes, such as the briefing note on stabilised approaches (see Guidance regarding stabilised approach criteria).
More recently, the FSF also developed a basic aviation risk standard (BARS) program that was designed to provide organisations that engaged contracted aircraft operators with a standard to assist in the risk-based management of aviation activities. It advised that the standard was suited to any organisation that used aircraft operators to provide contracted aviation support for its operations, particularly within remote and challenging environments. The program was used by several large mining/resource companies and other organisations. Participating aircraft operators could be audited against the standard.
The standard document stated:
All national and international regulations pertaining to aviation operations must be followed. This Standard is designed to supplement those requirements.
With regards to CFIT, the following risk controls were specified:
- 2 pilots for flights to be flown at night or ‘in IFR’
- multi-engine aircraft to be used for flights flown at night or ‘in IFR’
- aircraft operators to include type-specific stabilised approach requirements in their operations manual
- aircraft that fly under IFR or at night and on long-term contract to be fitted with an approved and serviceable Class A TAWS when an approved modification exists for the aircraft type (and the operator must have related procedures to be followed by the flight crew in the event of an alert).
The BARS implementation guide outlined guidance for stabilised approach criteria, based on the ALAR briefing note 7.1.
Operator’s CFIT risk mitigation
General aspects
At the time of the accident, Air Connect Australia was not required to have a safety management system (SMS). At the core of an SMS is a formal risk safety management process, which is used specifically to:
- identify hazards associated with an organisation’s operations
- analyse and assess the risks associated with those hazards
- implement control, to prevent future accidents, incidents or occurrences (CASA, 2018).
The operator advised it had an SMS incorporated into its operations manual, which included a requirement for 6-monthly safety meetings as well as a hazard, incident and accident report form. However, this did not include a detailed hazard identification and mitigation process. Without a documented hazard identification process, Air Connect Australia had not explicitly identified CFIT as a hazard nor detailed how it would mitigate the risk.
Based on the FSF CFIT checklist, the ATSB assessed that a single-pilot passenger transport operation involving non-precision approaches to airports without ATC and radar coverage (such as Lockhart River) in IMC carried a significant CFIT risk. Many of the risk-reduction factors listed in the checklist were specific to multi-crew operations and would not be appropriate or viable for a small operator conducting single-pilot operations such as Air Connect Australia. However, the checklist highlighted the CFIT risk for such an operation and provided some ways to mitigate the risk.
The ATSB further assessed Air Connect Australia and the aircraft (VH-OZO) against the suggested risk-reduction factors, as outlined in the following sections.
Section 1 – Company culture
The checklist items included aspects such as the operator placing safety before schedule and placing no negative conations on diversions or missed approaches. The chief pilot advised the ATSB that they often cancelled or postponed flights due to adverse weather. They also stated that they had frequent discussions with the pilot, and they were approachable and open to discussions with the pilot about any safety issues or concerns. Friends of the pilot were not aware of the pilot having any concerns about the chief pilot or the operator and confirmed that the pilot and chief pilot had frequent discussions about operational matters before flights.
Section 2 – Flight standards
The CFIT checklist did not specifically refer to stabilised approach criteria (which were introduced in later FSF guidance material). However, it listed a number of other items. Those potentially applicable to single-pilot operations included:
Reviewing approach or departure plates
Reviewing significant terrain along intended approach or departure course…
Briefing and observing MSA circles on approach charts as part of plate review
Checking crossing altitudes at IAF positions
Checking crossing altitudes at FAF and glideslope centering…
Use of 500-foot altitude call and other enhanced procedures for NPAs…
The operator’s operations manual stated, for all arrivals:
Before descending to an ALA [aircraft landing area], the Pilot-in-Command shall study available charts of the proposed ALA and surrounding area and make a note of hazards indicated on the charts.
The manual also required pilots to check the existing conditions in flight to determine their suitability for the approach and landing (including in terms of the MDA and required visibility on the approach charts). In addition, the manual included a requirement for crew to self-brief prior to the approach and also before landing:
Instrument Approaches – A self-briefing is to be carried out before every landing regarding the intentions of the Pilot-in-Command before / after the commitment point.
…
An emergency self-briefing is to be carried out before the landing regarding:
(a) the intentions of the Pilot-in-Command before / after the commitment point; and
(b) identifying missed approach track or heading.
As previously noted, the flight profile for a straight-in instrument approach also required a pilot to check the altimeter at the FAF. No other checks or altitude calls were explicitly stated.
Section 3 – Hazard awareness and training
The FSF checklist outlined various requirements for CFIT hazard awareness, including:
Your company’s pilots are reviewed annually about the following:
Flight standards operating procedures
Reasons for and examples of how the procedures can detect a CFIT “trap”
Recent and past CFIT incidents/accidents
Audiovisual aids to illustrate CFIT traps
Minimum altitude definitions…
The operator did not conduct formal hazard awareness training and there were no records of any training relating specifically to CFIT. There was no specific regulatory requirement for the operator to conduct such training.[72]
The checklist also included items regarding incident reporting and investigation:
You have an incident/exceedance review and reporting program
Your organization investigates every instance in which minimum terrain clearance has been compromised
The company culture items also referred to fostering a culture where CFIT incidents could be reported. The chief pilot advised they had frequent discussions with the pilot and believed there was a high level of mutual trust, such that that if the pilot had a concern they would feel comfortable coming to talk to the chief pilot about it. However, the chief pilot was not aware of the circumstances associated with the missed approach at Aurukun in January 2020.
The chief pilot advised that the pilot used their own tablet device (iPad) and OzRunways subscription. As a result, the chief pilot did not have access to the recorded data.
Section 4 – Aircraft equipment
The CFIT checklist referred to GPWS, radio altimeter, various types of displays and autopilot functions. The checklist was not up-to-date and not targeted to the needs and potential current opportunities for small aircraft.
As previously noted, VH-OZO was not fitted with a TAWS or radio altimeter, nor were these mandated by the regulatory requirements at the time. The aircraft’s GPS units did not provide vertical guidance for RNAV GNSS approaches and the autopilot was not capable of maintaining a vertical profile without pilot input.
CFIT risk score
Based on the total CFIT risk factors for the destination and risk multipliers, then consideration of the available risk-reduction factors, the total CFIT risk score calculated for the operator was less than zero and indicated a significant CFIT risk. However, as already noted, other operators conducting single-pilot charter flights involving non-precision approaches in IMC to airports without ATC and radar coverage (such as Lockhart River) would also be exposed to a significant CFIT risk as assessed by this checklist.
Safety analysis
Introduction
After conducting an area navigation (RNAV) global navigation satellite system (GNSS) approach to runway 30 at Lockhart River and then a missed approach, the pilot of the Cessna 404 aircraft (VH-OZO) immediately commenced a second RNAV GNSS approach to runway 30. The aircraft’s descent gradient was similar to the first approach but significantly lower than the recommended profile in the approach chart. This descent continued until the aircraft collided with terrain 6.4 km short of the runway.
There was no evidence of any conditions or circumstances likely to induce a medical problem or incapacitation for the pilot, who had been in good health and was well rested. Also, based on the recorded flight data and impact information, the aircraft appeared to be in controlled flight up until the time of the impact. Accordingly, it is very unlikely that the pilot was incapacitated or impaired during the flight.
There was no evidence of any aircraft system or mechanical anomalies that would have influenced the accident. However, as a consequence of extensive aircraft damage, it was not possible to be conclusive about the aircraft’s serviceability.
Therefore, based on the available evidence, the accident was very likely the result of controlled flight into terrain (CFIT). That is, an airworthy aircraft under the control of the pilot was flown unintentionally into terrain, probably with no (or very limited) prior awareness by the pilot of the aircraft’s proximity to terrain.
As evidenced in this case, a CFIT accident generally results in significantly adverse consequences for the occupants of an aircraft, and thus operators conducting operations in instrument meteorological conditions (IMC) or degraded visual conditions need to have robust risk controls to minimise the risk of such accidents.
This analysis considers the weather and sequence of events, followed by the factors that likely influenced the pilot’s performance. It also discusses risk controls for CFIT relevant to this accident.
Weather conditions
The weather conditions at Lockhart River at the time of the 2 approaches were consistent with the forecast, with periods of reduced visibility due to rain and cloud. The conditions had been suitable for the pilot of one aircraft to land about 1 hour prior to the accident and allowed another to land about 30 minutes afterwards.
Although the pilot of VH-OZO had not obtained the latest weather forecast for Lockhart River on the morning prior to the flight using their National Aeronautical Information Processing System (NAIPS) account, they could have obtained the forecast from other sources and they were reportedly aware of the current weather information. In any case, the pilot had ensured the aircraft had sufficient fuel to hold, divert or return to Cairns if necessary.
The landing minima for the runway 30 approach at Lockhart River included a cloud ceiling (for broken cloud or worse) of 730 ft and a visibility of 4,200 m. On arrival at Lockhart River, the pilot listened to the automated weather information service (AWIS) via VHF radio, and received information that the reported conditions were above the landing minima (with 10 km visibility and broken cloud at 1,800 ft).
The conditions recorded by the airport’s automatic weather station (AWS) at the time of the first approach’s final segment (0904–0907) included a visibility of 10 km, some rainfall (0.2 mm at 0904) and nil wind. The visibility was measured in the immediate vicinity of the sensor and may not have reflected the conditions experienced by the pilot during the approach. The recorded cloud base was 1,800 ft, however the recorded values for cloud were averaged over the preceding 30-minute period, and the cloud conditions at any specific time may have been worse than recorded.
Based on a witness report, it appeared as though the conditions at times were probably worse than recorded, with periods of reduced visibility due to cloud and rain. Messages sent by 2 of the passengers at 0914 indicated there was low visibility and one stated there was heavy rain, however it was not clear whether these comments were related to the conditions during the first approach or were observations of the conditions closer to 0914, which was about 7 minutes after the aircraft passed the missed approach point (MAPt).
Therefore, although the AWS observations indicated the weather was above the landing minima, there were areas of reduced visibility in the vicinity of the airport and it was not possible to conclusively determine the actual conditions experienced by the pilot when they reached the MAPt during the first approach.
The recorded visibility deteriorated to 800 m at 0912 and was below the landing minima for most of the period 0911–0917. This aligned with recorded rainfall between 0910–0916, including moderate to heavy rain during 0912–0914. Weather radar images from the Bureau of Meteorology (BoM) also showed rain passing through the Lockhart River area during both approaches, with heavier rain during the second approach. The radar images were consistent with the weather observations by people in the area, including that a ‘wall’ of heavy rain passed through at about the time of the accident. Given the direction the radar returns were moving, it is likely the aircraft entered the rain during the second approach.
In summary, while the pilot was operating in the vicinity of Lockhart River Airport, there were areas of cloud and rain that significantly reduced visibility and increased the risk of CFIT. In particular, the aircraft probably entered areas of significantly reduced visibility during the second approach. As a result of the reduced visibility, the pilot would have been reliant on the aircraft’s flight instruments and GPS units during both approaches.
Conduct of the approaches
First approach and missed approach
On the first approach, the pilot levelled at about 5,000 ft prior to the initial approach fix (IAF), which in this case was LHREB, then established the aircraft on a 3° descent from the IAF. This was consistent with the pilot’s normal method and conformed to the operator’s recommendations and the guidance shown on the approach chart. The pilot conducted the approach consistent with the recommended (3°) constant descent profile, and the aircraft kept descending through the minimum descent altitude (MDA) of 730 ft and passed the missed approach point (MAPt).
There were no significant lateral deviations from the published track, and the indicated airspeed when passing the final approach fix (FAF) was about 130 kt. However, after the FAF, the airspeed increased to about 140 kt, which was sustained throughout the remaining descent. This was significantly in excess of the operator’s preferred airspeed for such approaches (about 110 kt at the FAF), and it also exceeded the applicable handling speed limit specified in the Aeronautical Information Publication (AIP) of 130 kt in the final approach segment.
After passing the MAPt, the aircraft’s recorded descent rate was 900 to 960 ft/min. When the aircraft reached about 400 ft, the pilot initiated a missed approach. The airspeed at that time significantly exceeded the operator’s stabilised approach criteria speed (that is, about 110 kt at 300 ft above aerodrome elevation).
The reason for the non-conforming airspeed on this approach could not be determined. It is possible that this was intentional, and the pilot was expediting the arrival in response to the visible weather. Alternatively, it could have been inadvertent and associated with a focus on other flying tasks in this phase, such as maintaining track (with reduced tolerances) and/or searching for visual cues such as the runway threshold in reduced visibility (see also Awareness of procedural requirements). Whatever the reason, the high airspeed would have made it difficult to configure the aircraft for a stabilised final approach and landing.
Although the descent rate was just within the operator’s limit for a stabilised approach (that is, 1,000 ft/min), it was higher than the 600 ft/min that would be typical for this stage of a final approach. It was also higher than the 750 ft/min that would be commensurate with a 3° profile at 140 kt. Similar to the high airspeed, the reason for the relatively high descent rate could not be determined. It is possible that the pilot was attempting to maintain visual reference.
As previously noted, the weather conditions when the aircraft reached the MAPt could not be determined. It is possible that the conditions were better than the landing minima at that point but then deteriorated as the approach continued and when the aircraft was at a lower altitude.
Irrespective of the conditions, this was not a stable approach due to the relatively high airspeed and a missed approach was necessary. Ultimately, the pilot commenced the missed approach when the aircraft reached about 400 ft. Whether the decision to conduct the missed approach at that time was based on the weather conditions, airspeed, descent rate or some combination of those factors could not be determined.
After initiating the missed approach, the pilot was required to select one of 4 options:
- immediately conduct another instrument approach
- hold in the area, monitor the weather at Lockhart River and, if suitable, conduct another instrument approach
- divert to a nearby airport, monitor the weather at Lockhart River and, if suitable, return for another approach
- return to Cairns.
Having descended to 400 ft then overflown the airport during the missed approach, it is expected that the pilot had an appreciation of the low-level weather conditions. Then, during the missed approach, the aircraft tracked initially to the north-west, which was in the direction of weather that was moving towards Lockhart River. Given heavy rain was recorded at the airport about 5–7 minutes after the aircraft had been overhead, the boundary of this rain area may have been visible to the pilot. The rain should also have been displayed on the aircraft’s weather radar display and potentially also the iPad the pilot was using during the flight, although exactly what information was displayed to (or observed by) the pilot could not be determined.
As reported by a passenger in a text message at 0914, at some point during the missed approach or transition to the second approach, the aircraft was in or near heavy rain. Although the AWS between 0911 and 0917 was indicating below-minima weather at the airport, it is unlikely that the pilot had spare capacity to access the AWIS in that period.
Given this context, the pilot may have considered there was a window of opportunity to conduct a second approach before there was heavy rain at the airport and, accordingly, they expedited the second approach.
Second approach – vertical profile
The pilot tracked directly towards the IAF LHREA at 3,500 ft to commence the second approach. After passing the IAF, the aircraft remained at that altitude while heading to the IF. The pilot then commenced descent from 3,500 ft about 2.7 NM prior to the IF. From about 1.6 NM before the IF, the descent was flown at about a normal 3° flight path, although about 1,000 ft below the recommended descent profile. The aircraft descended through the intermediate segment minimum safe altitude of 1,800 ft and passed the FAF at about 1,100 ft. When the aircraft reached a recorded altitude of 700 ft, the descent rate increased from about 700 ft/min to about 1,200 ft/min until the collision with terrain.
The investigation considered 3 main scenarios to explain the vertical profile of the second approach. The first scenario is the pilot misunderstood their position along the approach (or misidentified the waypoints) and believed they were one segment (5 NM) further along the approach than they actually were. Figure 21 shows the vertical profile of the second approach (in red) and it also shows the vertical profile moved 5 NM to the left (in green), as if the pilot thought they were one segment further along the approach. With regard to this scenario:
- The scenario does not provide a good explanation of the recorded data as the aircraft remained about 600 ft above the recommended profile for an extended period and there was no indication of any attempt to correct such a perceived problem prior to reaching the perceived MAPt.
- The waypoint names on RNAV approaches are similar and there is some potential for confusion. However, in this case the pilot had significant cues to indicate their position along the approach, given they were turning at the IF (10 NM from the MAPt), and they had broadcasted they were at this position just prior to the turn. Although the absence of a subsequent broadcast by the pilot at the FAF (5 NM) could indicate a loss of awareness of the aircraft’s position at that time, it could also have been omitted because the pilot assessed it was unnecessary due to the lack of other aircraft in the vicinity, and/or the pilot was experiencing high workload.
Figure 21: Second approach to runway 30 (red), second approach displaced 5 NM (green) and second approached raised 1,000 ft (yellow)
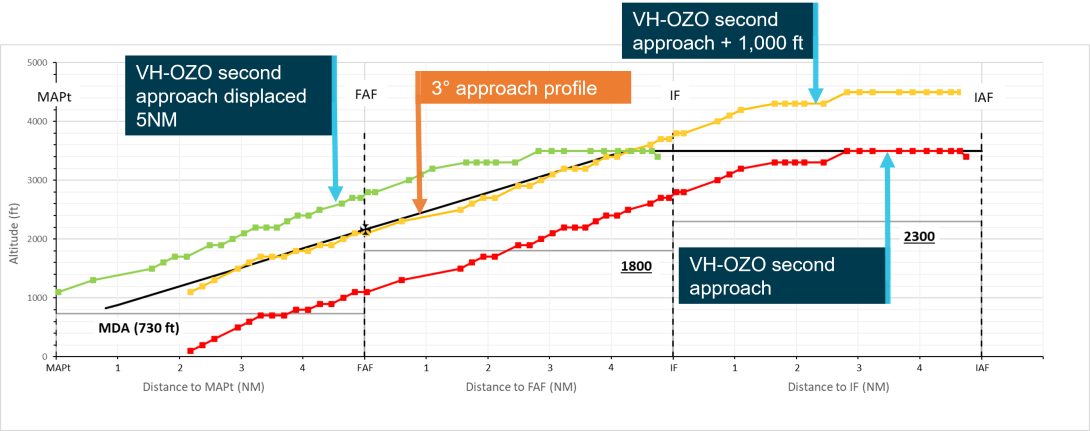
The second scenario is the pilot believed they were 1,000 ft higher than they actually were during most of the descent. This scenario is shown on Figure 21 (in yellow). With regard to this scenario:
- The scenario closely matches the recommended descent profile in terms of commencing the 3° descent at about the right point for a constant descent and then continuing the descent past the IF and FAF.
- When the pilot commenced the descent from 3,500 ft, they were correcting the aircraft’s lateral position (right of the initial approach track) and would therefore have been experiencing a high workload. The potential to mis-read instruments (such as an altimeter) is significantly increased under high workload, and the ability to subsequently detect and correct such an error would also be reduced, given that high workload can lead to scanning information sources less frequently and also scanning them for shorter durations.
- Just prior to passing the IF, the pilot broadcasted that they were at ‘3,800 correction 2,800 ft’, and this correction was an accurate broadcast of the aircraft’s altitude at the time. This indicated an initial mis-reading of the altimeter, but it also indicated that the pilot had correctly read the altimeter at that time. However, this correction occurred at one point in time for the purpose of making the radio broadcast, and the pilot may not have fully assimilated the information for the purpose of monitoring their descent profile.
- The pilot should have been regularly checking the altimeter during the descent as part of their instrument scan, so for this scenario to be viable the pilot would need to mis-read the altimeter multiple times, or at least not detect a problem when scanning the altimeter after an initial error. This would seem unlikely over an extended period. However, as noted before, the pilot may have been scanning instruments less frequently and for shorter durations due to workload. They may also have been focussing more on the vertical speed indicator than the altimeter after commencing the descent.
- The aircraft was fitted with a 3-pointer altimeter, which are widely used in small aircraft. Research has shown that pilots can mis-read this type of altimeter, including mis-reading by 1,000 ft, although accidents known to be associated with such errors seem relatively rare.
- The scenario does not specifically explain why the descent rate increased in the last 30 seconds of the approach. However, the pilot was probably experiencing a very high workload at that time associated with correcting the aircraft’s lateral position (see Second approach - lateral position). The pilot may also have started increasing the amount of time they were looking outside the aircraft for visual cues, and/or their attention was diverted when entering heavy rain. In addition, heavy rain on a windshield is known to create refraction effects that can lead a pilot to perceive that the aircraft is too high, which can result in an unwarranted nose-down correction and flight below the desired flight path (Flight Safety Foundation 2000).[73]
The third scenario is the pilot intentionally descended below the recommended descent profile and segment minimum safe altitude in order to maximise the chances of becoming visual before reaching the MAPt. For this type of scenario, it is unlikely a pilot would intentionally descend below the MDA before the MAPt unless there were some visual references. With regard to this scenario:
- There were no indications in the pilot’s recent RNAV GNSS approaches of descending this early on an approach or descending below segment minimum safe altitudes, although it is acknowledged that the pilot had limited experience with having to conduct a second approach in IMC. There was also no indication that the pilot took unnecessary risks in interviews with other pilots who had flown with the pilot.
- Lockhart River is a location widely known to be problematic in terms of the weather conditions and terrain. Although the pilot had flown to Lockhart River on several occasions, as far as could be determined they had not previously encountered IMC at this location before. Nevertheless, given the location’s reputation, it would be reasonable to expect that the pilot would be conducting approaches cautiously and therefore less likely to descend below altitude limits.
- If the pilot was intentionally descending early, it is not exactly clear why they would choose to conduct a 3° descent that was about 1,000 ft below the recommended descent profile. However, it is possible the pilot wanted to reach the MDA over water (to ensure more clearance from terrain) and had calculated that such a profile would enable the aircraft to be over water just after reaching the FAF.
- There was some indication in the recorded data that the aircraft’s descent rate may have briefly reduced near the MDA, with 3 successive data points recorded at 700 ft. This might be associated with an attempt to level out at about the MDA. Alternatively, the descent rate may have only briefly reduced from 700 ft/min to 500–600 ft/min due to the pilot’s workload and attention been focussed on the aircraft’s lateral position at that time, or due to the pilot’s attention being diverted when they entered heavy rain. It is also possible that the 3 data points at the same level were an artefact of the recorded data and there was no significant change in descent rate.
- If the pilot was attempting to level out at about 700 ft, it is unclear why the aircraft kept descending for over 30 seconds after reaching this altitude at a descent rate of 1,200 ft/min. It is possible the pilot did not retrim the aircraft after levelling out and then did not effectively monitor the altimeter due to other tasks, including looking outside to gain visual references.
In summary, following the missed approach, the pilot immediately conducted another approach to the same runway that was on a similar gradient to the recommended descent profile but displaced about 1,000 ft below that profile. While continuing on this descent profile, the aircraft descended below a segment minimum safe altitude and the minimum descent altitude, then kept descending until the collision with terrain about 6 km before the runway threshold.
Based on the available evidence, it is unlikely that the pilot thought they were one segment out on the approach and there was no specific evidence to indicate that the pilot had or would intentionally descend below the recommended descent profile and below a segment minimum safe altitude. Overall, mis-reading the altimeter by 1,000 ft appears to be the most likely scenario, although there was insufficient evidence to provide a definitive conclusion. Regardless of the exact scenario, it is evident from the continued descent that the pilot did not effectively monitor the aircraft’s altitude and descent rate for an extended period.
Second approach - lateral position
The aircraft was just within the capture region for the IAF LHREA when the pilot commenced the turn towards the IF, and it was then initially significantly to the right of the initial approach track. The pilot corrected the aircraft’s heading, but the aircraft was still more than half full-scale deflection on the course deviation indicator (CDI) at the time it started descending from 3,500 ft. The descent was paused soon after and then recommenced, before the IF, with the aircraft within the required half full-scale deflection in order to descend.
Before reaching the IF, the aircraft was close to the initial approach track. The pilot then turned slightly late at the IF and the aircraft was left of the intermediate approach track, reaching about half full-scale deflection on the CDI. The pilot promptly took corrective action, though the aircraft then started deviating to the right of the approach track about 3 NM prior to the FAF. However, the lateral deviation at that stage was less than half full-scale deflection.
Nearing the FAF, the sensitivity of the CDI increased, and when the aircraft passed the FAF it was at about full-scale deflection on the CDI (as presented by the Garmin GNS 430W), and then it exceeded full-scale deflection for about 55 seconds (and was outside half-scale deflection for about 60 seconds). The aircraft’s change of track to the left during the final 30 seconds of the approach suggests the pilot had observed the CDI and attempted to correct the situation, but had then flown through the final approach track and, before a further correction back to the right could be conducted, the aircraft impacted terrain.
Given the aircraft was more than half full-scale deflection on the CDI when it reached the FAF, the pilot was required to conduct a missed approach in accordance with the operator’s stabilised approach procedures. It is acknowledged that the GNS 430W presented full-scale CDI deflection more restrictively than the required navigation performance (RNP) requirements specified in Civil Aviation Order (CAO) 20.91 (that is, 0.23 NM compared to the RNP of 0.3 NM at the FAF). Nevertheless, given that the aircraft’s position was at full-scale deflection (rather than half-scale deflection), this difference should not have affected the pilot’s decision.
In addition, the aircraft was at 0.3 NM lateral displacement shortly after passing the FAF (for about 10 seconds). This would have been displayed to the pilot as more than full-scale CDI deflection, and the lateral displacement distance would also have been displayed on the graphical CDI on the default NAV page of the GNS 430W. Accordingly, the pilot was also required to conduct a missed approach in accordance with the CAO 20.91 requirements at that time.
Finally, with reference to the AIP, the pilot was also required to be within half full-scale deflection on the CDI after passing the FAF before continuing the descent below the previous segment minimum safe altitude (1,800 ft). This should have precluded further descent when passing the FAF (actual altitude 1,100 ft) or soon after (when the actual height was 800 ft and the perceived height may have been 1,800 ft).
It is possible the pilot may not have promptly detected the lateral deviation due to other workload, or they may not have fully realised the extent of the change in the sensitivity of the CDI near the FAF. Alternatively, it is possible that the lateral deviation was due, in part, to the pilot attempting to avoid the most adverse weather.
In summary, the pilot experienced some difficulty controlling the aircraft’s lateral position throughout the second approach. In particular, when passing the FAF and after passing the FAF, the aircraft reached or exceeded the required limits to conduct a missed approach, and a missed approach was not conducted. Regardless of the reason for the lateral deviations, by continuing the approach the pilot significantly increased their workload and the risk of collision with terrain/obstacles.
Second approach - indicated airspeed
The aircraft was at about 135 kt when it passed the FAF, before increasing to 140 kt soon after the FAF. It then increased to 150 kt towards the end of the flight, which was associated with the increased descent rate.
By exceeding the operator’s preferred speed of 110 kt at the FAF, the pilot significantly increased their workload and reduced the time available in the final approach segment to detect and identify other problems with the conduct of the approach (in terms of altitude, descent rate or lateral deviation). More specifically, if the airspeed had been about 110 kt at the FAF, and remained at or below that speed, the pilot would have had over 20 seconds more time prior to the collision to identify problems with the aircraft’s flight path (as well as additional time prior to reaching the FAF). A lower speed would also have made it easier to effectively correct the aircraft’s lateral position.
Unstable approach
Overall, at times during the conduct of the second approach, the pilot appeared to experience difficulty controlling the aircraft’s lateral position, and after passing the FAF they did not effectively control the aircraft’s altitude, descent rate, lateral position and airspeed. As already noted, the approach did not meet the operator’s stabilised approach criteria for lateral deviation at the FAF, and the pilot was required to conduct a missed approach at that point.
In addition, the second approach did not meet the stabilised approach criteria for airspeed (110 kt maximum) or descent rate (1,000 ft/min) at the operator’s applicable height of 300 ft above aerodrome elevation. At this point, the aircraft was travelling at about 150 kt and descending at 1,200 ft/min. However, given that the pilot may have believed the aircraft was 1,000 ft higher than it actually was during the approach, or may have thought they were in level flight near the MDA, they may have not yet considered these stabilised approach criteria.
Factors influencing pilot performance
Introduction
As previously noted, there was no evidence to indicate that the pilot was incapacitated during the accident flight. There was also no evidence to indicate the pilot was experiencing fatigue. The investigation considered a range of other factors that could have influenced the pilot’s performance, including workload, instrument flying proficiency, awareness of procedural requirements, and operational, social or organisational pressures.
Workload and monitoring
Single-pilot operations under the instrument flight rules (IFR), conducting instrument approaches in poor visibility and hand flying an aircraft are all demanding tasks. A pilot needs to be regularly scanning and interpreting the flight instruments, GPS unit (or navigational display) and approach chart, and assimilating the information from multiple sources. As they near the MAPt, they also need to be looking outside to evaluate the visual conditions.
It could not be determined with certainty whether the pilot was using a paper approach chart clamped to the control column and/or was referring to an iPad with the approach chart displayed. Based on the evidence available, it was more likely the pilot was using an iPad, but it was not clear whether it was secured to the control column or if it was on the pilot’s knee. If they were routinely scanning an iPad that was not fixed to the control column, this would have increased the difficulty of the pilot’s scanning and exacerbated the inherent workload associated with the tasks.
Overall, the pilot’s workload on the second approach was elevated throughout the approach compared to the first approach (and other recent instrument approaches conducted by the pilot). Initially the workload was elevated because they joined the approach directly after conducting the missed approach and from just within the capture region of the IAF LHREA. This limited their time to prepare for the approach, and also required more manoeuvring than normal at the IAF.
After turning at the IAF, the pilot’s workload was further elevated by the need to correct the aircraft’s track while also reducing airspeed. As previously noted, it was during this period that the pilot decided to commence the descent from 3,500 ft, which also involved selecting the approach flap and lowering the landing gear soon after.
The pilot’s workload after turning at the IF was also elevated due to the turn being slightly late and then needing to correct the aircraft’s track. This workload would have been significantly elevated after passing the FAF, with the deviation from the final approach track needing more significant correction, as well as due to potentially starting to look more outside the aircraft to assess the visual conditions.
At some point the aircraft probably entered rain, and this would also have increased the pilot’s workload and the difficulty of their task. The presence of rain decreases contrast in the visual environment, making it more difficult to detect terrain features. This effect is exacerbated when approaching or flying over water. In addition, as already discussed, heavy rain on a windshield can lead to a perception of being too high. Light rain can also result in a pilot misperceiving their aircraft to be higher than it is, resulting in a tendency to pitch down (Gibb and others 2010, Flight Safety Foundation 2000b, Previc 2004).
A further factor potentially increasing the difficulty of the pilot’s task after passing the FAF was the increasing sensitivity of the Garmin GNS 430W’s CDI. At and just past the FAF, where a decision to conduct a missed approach should have been made, full-scale deflection on the 430W was not significantly different to the RNP. Towards the MAPt, the 430W was significantly more restrictive than the RNP. The pilot should have been familiar with the system, although the extent to which they fully appreciated the effect of the increasing sensitivity could not be determined.
As previously noted, the omission of the broadcast at 5 NM could be an indication of the pilot’s high workload at that time. In addition, the aircraft’s increased descent rate and speed in the final 30 seconds as the pilot was correcting the lateral deviation is also consistent with the effects of high workload, reducing the ability to monitor and effectively control multiple flight parameters at the same time.
As previously discussed, the pilot’s workload could have been reduced by conducting the approach at a lower airspeed prior to and after the FAF. In addition, there were other available options after conducting the missed approach, including taking more time prior to conducting the second approach and commencing it from another position (for example, tracking further away from the IAF and then approaching it from closer to the centre of its capture region). Alternatively, the pilot could have conducted a holding pattern and waited for the weather to pass before attempting the second approach.
Such options would have provided more time to prepare for the approach and minimise the potential for altitude, descent rate or lateral deviations or other workload problems. These options may also have provided the pilot more opportunity to use the autopilot to reduce their sustained workload prior to initiating the second approach.
Instrument flying proficiency
Many pilots experience some difficulty with developing instrument flying skills and then consistently maintaining those skills. The pilot of the accident flight experienced some difficulties obtaining their initial instrument rating in 2014, and also experienced some difficulties in subsequent instrument proficiency checks (IPCs) in 2016 (underpinning knowledge) and 2017 (mis-reading the altimeter), before passing subsequent assessments. The pilot also experienced significant difficulties in conducting instrument approaches, including the management of multiple flight parameters at the same time, when being assessed in an airline simulator in early 2018, albeit in a much different environment to their previous operations.
The pilot’s last IPC was conducted in August 2019, 7 months prior to the accident, and no problems were noted. However, on that occasion, the Cessna 310 aircraft used for the check was fitted with a navigational system capable of providing vertical guidance for an RNAV GNSS approach. As far as could be determined, the pilot’s instrument flying skills using VH-OZO (a Cessna 404 with GNS 430 GPS units) or similar aircraft had not been observed by the chief pilot or a flight examiner pilot since they were cleared for line operations in October 2018.
Since their last IPC, the pilot had regularly logged RNAV GNSS approaches in VH-OZO and these approaches were generally flown accurately. However, these approaches were almost all conducted in VMC, which would have provided the pilot with cues to identify and correct problems with the aircraft’s position.
The pilot had only conducted one previous approach since the last IPC that potentially encountered IMC for a brief period, at Aurukun on 22 January 2020. This approach was not initiated in the pilot’s normal manner (with a descent from about 5,000 ft at the IAF). In addition, the aircraft reached the MDA 200–300 ft below the recommended descent profile and there were problems with speed control, with the speed after the FAF reaching 145 kt.
Overall, it was difficult to assess the pilot’s instrument flying skills at the time of the accident. Given the limited number of recent RNAV GNSS approaches in IMC or simulated IMC, it is conceivable that the pilot would have experienced significant workload in conducting an approach in IMC, particularly in circumstances where the approach required them to use a different method to normal. They would probably also have had limited ability to manage any additional problems or tasks that occurred during the conduct of an approach in IMC.
Awareness of procedural requirements
The operator’s published flight profile for an RNAV GNSS approach required a Cessna 404 to be at an indicated airspeed of about 90–100 kt at the FAF. However, interviews with the operator’s relevant personnel suggested that the operator’s preferred speed was probably about 110 kt. Given that the one-engine inoperative best rate of climb speed for the aircraft type was 102 kt (flap in the take-off/approach position and gear up), 110 kt was a more appropriate speed at the FAF. The pilot’s approaches routinely exceeded the operator’s preferred speed by a significant margin, with speeds of 130–140 kt being common.
Although the pilot should have been familiar with the contents of the operations manual, it is unclear to what extent they were aware of the flight profile speed or the operator’s preferred speed, and, if they were aware, the extent to which they thought these were reasonable expectations. In other regards, the pilot appeared to be conducting RNAV GNSS approaches in a manner consistent with the chief pilot’s expectations, using a constant descent from about 5,000 ft at the IAF in order to maximise the potential for a stabilised approach.
Having held an instrument rating since 2014, and been subject to many IPCs since then, the pilot should have been aware of the requirement to be to be no faster than 130 kt after the FAF while conducting an RNAV GNSS approach. They should also have been aware of the operator’s requirement to be within half-scale deflection of CDI in the final approach segment.
There were no indications from interviews with various people who flew with the pilot to suggest that the pilot routinely or intentionally deviated from procedural requirements. Given many of the pilot’s previous instrument approaches were conducted in VMC, it is possible the pilot did not consider that some procedural requirements, such as keeping the speed below 130 kt, were essential during such approaches. The pilot may have then become familiar with using such speeds and, when actually conducting approaches in IMC, did not fully recognise the problem. Alternatively, as already discussed, these speed deviations on the approaches in IMC may have been unintentional and were symptoms of high workload and/or limited proficiency.
Operational, social and organisational pressures
Pilots conducting passenger charter flights can experience or perceive a range of social and organisational pressures that can influence the potential to conduct or continue flights in unsuitable conditions (Paletz and others 2009, Michalski and Bearman 2014). These can include not wanting to disappoint customers, reluctance to admit defeat or time-related pressures.
Although such pressures can exist in operational environments, the ATSB identified no evidence to indicate the pilot was subject to any pressure to conduct this flight, complete the flight as quickly as possible, or to deviate from procedural requirements. The chief pilot had previously cancelled flights due to poor weather, but the forecast conditions in this case did not necessitate that the flight be cancelled.
As previously noted, the pilot had ensured the aircraft had sufficient fuel to conduct holding after arrival at Lockhart River, and divert or return to Cairns. They had also conducted holding for an extended period after a missed approach on a previous flight to Aurukun while waiting for a storm to pass, without any apparent negative social or organisational consequences.
As noted above, it is possible that, following the missed approach during the accident flight, the pilot assessed that the weather conditions were worsening and they had limited time to conduct a second approach before a more extensive delay. It is also possible that the pilot was worried about some aspect of the aircraft’s serviceability and did not want to delay the flight further. However, as previously noted, there was no evidence available to indicate a problem with the aircraft’s serviceability.
The pilot had previously commented to a passenger that they would only ever attempt 2 approaches prior to diverting. To what extent this may have placed self-imposed pressure on the pilot on this occasion to continue the approach could not to be determined.
Summary
In summary, the pilot was probably experiencing a very high workload during periods of the second approach. In addition to the normal high workload associated with a single pilot hand flying an approach in IMC, the pilot’s workload was elevated due to conducting an immediate entry into the second approach, conducting the approach in a different manner to their normal method, the need to correct lateral tracking deviations throughout the approach, and higher than appropriate speeds in the final approach segment. The workload was potentially further exacerbated by the pilot having limited recent experience in conducting RNAV GNSS approaches in IMC.
There was no evidence to indicate any organisational or commercial pressures on the pilot to complete the flight, but the extent to which self-imposed pressures or incomplete knowledge of procedural requirements influenced the pilot’s performance could not be reliably determined.
Risk controls for minimising the likelihood of controlled flight into terrain
Introduction
CFIT accidents have been a significant problem over the years, although the rate of such accidents has been decreasing. In Australia, the number of CFIT accidents during instrument approaches decreased from 9 in the 10-year period 1996–2005 and only 1 in the subsequent 15 years (that is, the accident involving VH-OZO at Lockhart River).
This decrease can be attributed, at least in part, to improved instrument approach designs, the increased use of better equipment to display relevant lateral and vertical navigational information, the increased fitment of a terrain warning system (TAWS), and greater use of constant descent angle approaches. However, risk factors still remain, particularly for smaller operators.
Air Connect Australia was a very small operator, with effectively only 2 pilots and one conducting almost all of the operator’s flights. Nevertheless, it conducted passenger transport operations in an aircraft with up to 9 passengers, and multiple factors existed that indicated a significant CFIT risk. These factors included:
- single-pilot operations under the IFR
- many of the operator’s destination aerodromes were in uncontrolled airspace and outside radar coverage
- most of the destination aerodromes offered only non-precision approaches with limited runway lighting systems and without visual approach slope indicating systems
- some of the destination aerodromes (such as Lockhart River) were associated with frequent rainfall or adverse weather conditions.
Although the operator had started introducing a safety management system (SMS), it had not developed a formal hazard register and a formalised approach to addressing the risk of hazards such as CFIT. The operator had introduced various measures to manage CFIT risk, such as a flight profile and stabilised approach criteria. However, there were limitations with these and some of the operator’s other risk controls.
Design of the flight profile
The operator’s operations manual included a flight profile for RNAV GNSS and other straight-in runway approaches. Such a flight profile can be an effective way of summarising an operator’s requirements and expectations for pilots regarding how an approach should be conducted.
However, as previously noted, the stated speed on the profile at the FAF (Vref to Vref + 10 kt) was very conservative and unrealistic in most situations. In addition, it did not appear to reflect the expectations of the operator’s key personnel (which equated to Vref + 20 kt or about 110 kt). The flight profile also did not include any requirements or expectations at the IF, which increased the potential for ambiguity.
Design of stabilised approach criteria
An essential and effective means of minimising the risk of CFIT accidents is for an operator to publish clear and relevant stabilised approach criteria, and then ensure that flight crew are aware of and comply with these criteria. Air Connect Australia’s stabilised approach criteria covered relevant parameters and factors, such as airspeed, configuration, descent rate, lateral tracking and briefings/checklists. The chief pilot also reportedly promoted the use of stabilised approaches to the pilot.
However, other than for lateral flight path deviations, the operator’s published criteria had an applicable height of 300 ft above aerodrome elevation for operations in both IMC and VMC. Such a height will have limited effectiveness for non-precision (or 2D) instrument approaches conducted in IMC, particularly given that the MDA for such approaches will often be above 300 ft. An applicable height of 1,000 ft above aerodrome elevation in IMC is widely recommended, including for operations in small aeroplanes. This will enable pilots to ensure that an approach is stable well before reaching the MAPt, reducing workload and maximising the time available to monitor the approach and identify and correct any problems.
The pilot of the accident flight generally appeared to be close to the stabilised approach speed of 110 kt at 300 ft in most of the instrument approaches examined by the ATSB, with 2 main exceptions being the first approach at Aurukun on 22 January and the first approach at Lockhart River, both of which resulted in missed approaches. Based on this information, the pilot appeared to be generally complying with the stabilised approach criteria. Therefore, had the operator specified an applicable height of 1,000 ft in IMC, it seems likely that the pilot would generally have been conducting approaches with a lower speed at this height.
However, it is not clear whether an applicable height of 1,000 ft would have influenced the pilot’s performance and changed the outcome of the second approach on the accident flight. As previously discussed, the exact reasons why the aircraft was descending below the recommended descent profile could not be determined. The pilot may have believed the aircraft was 1,000 ft higher than it was, and therefore thought the aircraft was still above 1,000 ft in the period immediately prior to the collision. Nevertheless, if they were effectively monitoring the flight instruments and complying with the criteria, they should have been slowing down well before reaching an altitude of 1,100 ft (the applicable height at Lockhart River given the aerodrome elevation).
An applicable stabilised approach height of 1,000 ft has been widely recommended by organisations such as the International Civil Aviation Organization (ICAO), the Flight Safety Foundation (FSF) and overseas regulators. However, as of the time of the accident, no formal guidance regarding the content of stabilised approach criteria had been published for Australian operators by the Civil Aviation Safety Authority (CASA). The ATSB has identified a number of Australian operators over the years conducting passenger transport operations under the IFR with applicable heights for all approaches of 300 ft. Accordingly, providing more specific formal guidance in Australia should reduce the likelihood that other operators will use such heights as the reference point for their criteria for all approaches.
It is acknowledged that operators need to tailor stabilised approach criteria to their specific aircraft and operations, and the use of 300 ft may be appropriate for some criteria on some types of approaches. In particular, circling approaches could involve a turn onto final approach at about 500 ft, and therefore cannot meet all stabilised approach criteria until about 300 ft (which is acknowledged in the FSF’s recommended criteria). In addition, it may not be appropriate to select full flap on many smaller aeroplanes until much lower than 1,000 ft on an approach (although other criteria could be met at a different height).
Nevertheless, with greater use of straight-in approaches, all operators should ensure the applicable heights for their stabilised approach criteria are consistent with contemporary guidance where possible for such approaches, particularly for operations in IMC.
Fitment of a terrain avoidance and warning systems
The use of a terrain avoidance and warning system (TAWS) has been shown to significantly reduce the risk of a CFIT. However, VH-OZO was not fitted with a TAWS, nor was one required to be fitted.
Given the aircraft’s descent profile on the second approach, if a TAWS had been fitted and been operational, it would have provided the pilot with both visual and aural alerts of the approaching terrain for an extended period. Accordingly, it is very likely that the accident would not have occurred.
The requirements for a TAWS have been increasing over time. However, the requirements in Australian have lagged behind those in comparable countries, and they were also not consistent with ICAO standards and recommended practices. In Australia there was a requirement for turbine-engine aeroplanes carrying 10 or more passengers on air transport flights under the IFR to be fitted with a TAWS, whereas the ICAO standard required aeroplanes authorised to carry 10 or more passengers to be fitted with a TAWS, regardless of the number of passengers carried on a flight. More importantly:
- There was no requirement for piston-engine aeroplanes conducting air transport operations to be fitted with a TAWS, even though this had been an ICAO standard since 2007 for such aeroplanes authorised to carry 10 or more passengers, and this standard had been adopted as a requirement in many comparable countries.
- There was no requirement for turbine-engine aeroplanes conducting air transport operations that were authorised to carry 6–9 passengers to be fitted with a TAWS, even though this had been an ICAO recommended practice since 2007, and this recommended practice had been adopted as a requirement in many comparable countries.
CASA had been consulting on applying TAWS requirements to more aircraft since 2008, and had proposed introducing a TAWS requirement for aeroplanes conducting air transport operations (turbine- and piston-engine) with a maximum operational passenger seat configuration (MOPSC) of 6 or more in 2012. However, following consultation with industry, this proposal was amended in 2018 to only require aeroplanes with a MOPSC of 10 or more to be fitted with a TAWS, with the application date being December 2021 or December 2022 depending on various factors.
VH-OZO had 12 seats that could be used by passengers and therefore it had a MOPSC of 12 when used for single-pilot operations. If it remained in that configuration, the operator would have had to have fitted a TAWS to the aircraft by December 2022 and, by December 2024, operated the aircraft under CASR Part 121 (which imposed additional requirements, including 2 pilots for all flights). Given that context, it is likely that an operator in this situation would have chosen to modify such an aircraft so that its MOPSC was 9 or less. Consequently, even if the changes to the TAWS requirements had been introduced in Australia earlier, they probably would not have resulted in an aircraft such as VH-OZO being fitted with a TAWS.
In terms of other comparable countries, only Canada has introduced a requirement for piston-engine aeroplanes with a passenger seating capacity of 6–9 seats to be fitted with a TAWS. However, it is noted that Canada generally experiences more adverse weather conditions than most areas of Australia.
In summary, there was no requirement for VH-OZO to be fitted with a TAWS, and piston-engine aeroplanes with a passenger seating capacity of 6–9 seats were not required to have a TAWS in most comparable countries. Accordingly, it would probably have been difficult to justify mandating that such aeroplanes be fitted with a TAWS in Australia.
Nevertheless, the lessons from the VH-OZO accident (and many previous accidents) are clear: all operators conducting passenger transport operations under the IFR in aircraft that do not currently have a TAWS should recognise the substantial benefits of a TAWS, and be actively seeking to install a TAWS in their aircraft to maximise the safety of their operations.
Use of the GNS 430W terrain awareness function
Although not developed to the same standard and with the same functionality as a TAWS, the Garmin GNS 430W units fitted to VH-OZO had a terrain awareness function that was capable of providing visual pop-up terrain alerts. If the function was selected on, then the system should have displayed a terrain alert to the pilot within the last 30 seconds of the flight (and potentially longer).
However, the terrain awareness function could be inhibited by a pilot, in which case no terrain alerts would be displayed. It could not be determined whether the function was selected or inhibited during the approaches at Lockhart River.
Given the pilot had significant experience using GPS units with a terrain awareness function, it would be expected that they were aware of the function and had seen terrain alerts in the past. However, the investigation could not determine how the pilot normally used the function (that is, whether they normally had it selected on or inhibited).
The Garmin 400W Series Pilot’s Guide & Reference manual explicitly stated that the system’s terrain awareness function was to be used for supplemental awareness only and was not to be relied upon for terrain avoidance. Nevertheless, although not as effective as a TAWS, the terrain awareness function could still be a valuable tool during instrument approaches in IMC if an operator (and its pilots) had clearly-defined procedures, training and guidance on how to effectively and safely use the function.
For example, an operator could require its pilots to confirm that the function was selected on as part of the descent checklist (particularly when conducting an instrument approach). However, the operator of VH-OZO did not have any explicit procedures or guidance, and this was similar to other operators who used the same type of equipment.
Vertical guidance information
At the time of the accident, the approaches available to the pilot at Lockhart River were RNAV GNSS approaches available for runways 12 and 30, and an NDB approach for runway 30. These were non-precision approaches that did not provide vertical guidance to pilots.
More advanced GPS/navigational systems than the GNS 430W, such as that fitted to the aircraft in which the pilot conducted their most recent IPC, can provide vertical guidance to aid in maintaining the correct vertical profile for a non-precision RNAV GNSS approach.
Vertical guidance information (showing the aircraft’s vertical position during the approach) would have significantly assisted the pilot in maintaining awareness of the aircraft’s vertical position and would have provided a salient indication that the aircraft was dangerously low for an extended period. Having vertical guidance available would also have reduced the pilot’s workload, such as reducing the extent of the mental calculations required to ascertain the aircraft’s position versus altitude and any necessary corrections to the descent rate.
There was no requirement for VH-OZO to be fitted a GPS/navigational system that provided vertical guidance information. However, in the absence of a TAWS, such a system could have significantly reduced the risk of CFIT during instrument approaches.
Monitoring of line operations
The pilot generally conducted instrument approaches in a manner that was consistent with the chief pilot’s preferred method. However, the approaches were also conducted at speeds significantly higher than the operator’s preferred speed. Although they were having regular discussions with the pilot about their flights, the chief pilot had no awareness that this was occurring.
One means of identifying potential problems with the conduct of line operations is through regular proficiency checks. The pilot had not received an operator proficiency check (OPC) since being checked to line in October 2018, and the chief pilot (or the designated flight examiner who could conduct OPCs) had also not flown with the pilot since that time. The pilot had completed an IPC in August 2019, although with a flight examiner who was not familiar with the operator’s procedures and in a different aircraft, with different navigational equipment, than the operator used.
At the time of the accident, there was no specific regulatory requirement for most charter operators to conduct OPCs on their pilots (though pilots conducting IFR operations still needed to undertake an IPC every 12 months). However, as of December 2021, such operators needed to conduct OPCs (or flight crew member proficiency checks) every 6 months.
It is acknowledged that OPCs will not necessarily identify all problems, and pilots may sometimes perform differently when being observed compared to when they are not being observed. However, such checks provide a valuable opportunity for identifying potential misunderstandings regarding the operator’s expectations about how key aspects of flight operations should be conducted.
Another option now readily available to small operators is the use of GPS-based flight data, either through an electronic flight bag application (EFB) or via other sources. Such data may only include some basic flight parameters, which is not as useful as a flight data recorder or other device specifically designed for monitoring flight operations. However, it could still assist with evaluating some aspects of flight operations, including the way instrument approaches are conducted.
Reviewing recorded GPS-based flight data would require a chief pilot or other designated person to be aware of the limitations of the data, and the process would be best done cooperatively with pilots. Nevertheless, the process would not require significant resources and, if done on a regular basis, could help identify potential misunderstandings regarding the operator’s expectations and also provide useful feedback and learning opportunities for pilots.
Hazard awareness training
As stated by the FSF, another useful mitigator for reducing the risk of CFITs is hazard awareness training. The operator had not developed any hazard awareness training material relating to CFIT, and it would be difficult for a small operator to develop a detailed and tailored training program. Nevertheless, various resources from the FSF could be selected and used to provide some guidance information to pilots, together with focussed discussions regarding flight profiles and stabilised approach criteria.
Summary
Ideally, in order to minimise the risk of CFIT, operators conducting passenger transport operations under the IFR would use aircraft fitted with a TAWS and/or have a GPS/navigational system that provides vertical guidance during a non-precision instrument approach. However, without such equipment being a regulatory requirement, it would be difficult for some operators to justify the cost, including in cases where they are leasing the aircraft from another organisation (as was the case with Air Connect Australia).
Nevertheless, there are other means available for such operators to minimise CFIT risk. In this case, the operator had included stabilised approach criteria and flight profiles in its operations manual, and it encouraged the use of stabilised approaches. However, there were problems with the applicable height for the criteria (that is, not 1,000 in IMC) and the flight profile for straight-in approaches did not fully reflect the operator’s requirements or preferences. The operator had also not developed clear procedures and guidance for the use of the terrain awareness function in the aircraft’s 430W GPS/navigational units, and had not conducted regular OPCs or other checks of line operations. Overall, improving these procedural controls would have reduced the operator’s CFIT risk and probably reduced the likelihood of this particular accident.
It is likely that many of these limitations will also exist in other small operators conducting passenger transport operations in small aircraft. Accordingly, this accident has provided many important lessons to such operators regarding ways they can minimise their CFIT risk during IFR operations. These are summarised in the Executive summary.
Findings
ATSB investigation report findings focus on safety factors (that is, events and conditions that increase risk). Safety factors include ‘contributing factors’ and ‘other factors that increased risk’ (that is, factors that did not meet the definition of a contributing factor for this occurrence but were still considered important to include in the report for the purpose of increasing awareness and enhancing safety). In addition ‘other findings’ may be included to provide important information about topics other than safety factors. Safety issues are highlighted in bold to emphasise their importance. A safety issue is a safety factor that (a) can reasonably be regarded as having the potential to adversely affect the safety of future operations, and (b) is a characteristic of an organisation or a system, rather than a characteristic of a specific individual, or characteristic of an operating environment at a specific point in time. These findings should not be read as apportioning blame or liability to any particular organisation or individual. |
From the evidence available, the following findings are made with respect to the controlled flight into terrain involving a Cessna 404 aircraft, registered VH-OZO, which occurred near Lockhart River Airport, Queensland, on 11 March 2020.
Contributing factors
- While the pilot was operating in the vicinity of Lockhart River Airport, there were areas of cloud and rain that significantly reduced visibility and increased the risk of controlled flight into terrain. In particular, the aircraft probably entered areas of significantly reduced visibility during the second approach.
- After an area navigation (RNAV) global satellite system (GNSS) approach to runway 30 and missed approach, the pilot immediately conducted another approach to the same runway that was on a similar gradient to the recommended descent profile but displaced about 1,000 ft below that profile. While continuing on this descent profile, the aircraft descended below a segment minimum safe altitude and the minimum descent altitude, then kept descending until the collision with terrain about 6 km before the runway threshold.
- Although the exact reasons for the aircraft being significantly below the recommended descent profile and the continued descent below the minimum descent altitude could not be determined, it was evident that the pilot did not effectively monitor the aircraft’s altitude and descent rate for an extended period.
- When passing the final approach fix (FAF), the aircraft’s lateral position was at about full-scale deflection on the course deviation indicator (CDI), and it then exceeded full-scale deflection for an extended period. In accordance with the operator’s stabilised approach procedures, a missed approach should have been conducted if the aircraft exceeded half full-scale deflection at the FAF, however a missed approach was not conducted.
- The pilot was probably experiencing a very high workload during periods of the second approach. In addition to the normal high workload associated with a single pilot hand flying an approach in instrument meteorological conditions, the pilot’s workload was elevated due to conducting an immediate entry into the second approach, conducting the approach in a different manner to their normal method, the need to correct lateral tracking deviations throughout the approach, and higher than appropriate speeds in the final approach segment.
- The aircraft was not fitted with a terrain avoidance and warning system (TAWS). Such a system would have provided visual and aural alerts to the pilot of the approaching terrain for an extended period, reducing the risk of controlled flight into terrain.
- Although the aircraft was fitted with a GPS/navigational system suitable for an area navigation (RNAV) global satellite system (GNSS) approach and other non-precision approaches, it was not fitted with a system that provided vertical guidance information, which would have explicitly indicated that the aircraft was well below the recommended descent profile.
- Although the operator had specified a flight profile for a straight-in approaches and stabilised approach criteria in its operations manual, and encouraged the use of stabilised approaches, there were limitations with the design of these procedures. In addition, there were limitations with other risk controls for minimising the risk of controlled flight into terrain (CFIT), including no procedures or guidance for the use of the terrain awareness function on the aircraft’s GNS 430W GPS/navigational units and limited monitoring of the conduct of line operations. (Safety Issue)
Other factors that increased risk
- Although an applicable height of 1,000 ft for stabilised approach criteria in instrument meteorological conditions has been widely recommended by organisations such as the International Civil Aviation Organization for over 20 years, the Civil Aviation Safety Authority had not provided formal guidance information to Australian operators regarding the content of stabilised approach criteria. (Safety issue)
- The Australian requirements for installing a terrain avoidance and warning system (TAWS) were less than those of other comparable countries for some types of small aeroplanes conducting air transport operations, and the requirements were not consistent with International Civil Aviation Organization (ICAO) standards and recommended practices. More specifically, although there was a TAWS requirement in Australia for turbine-engine aeroplanes carrying 10 or more passengers under the instrument flight rules:
- There was no requirement for piston-engine aeroplanes to be fitted with a TAWS, even though this was an ICAO standard for such aeroplanes authorised to carry 10 or more passengers, and this standard had been adopted as a requirement in many comparable countries.
- There was no requirement for turbine-engine aeroplanes authorised to carry 6–9 passengers to be fitted with a TAWS, even though this had been an ICAO recommended practice since 2007, and this recommended practice had been adopted as a requirement in many comparable countries. (Safety Issue)
Other findings
- The forecast weather at Lockhart River for the time of the aircraft’s arrival required the pilot to plan for 60 minutes holding or diversion to an alternate aerodrome. The aircraft had sufficient fuel for that purpose; and the aircraft had sufficient fuel to conduct the flight from Cairns to Lockhart River and return, with additional fuel for holding on both sectors if required.
- There was no evidence of any organisational or commercial pressure to conduct the flight to Lockhart River or to complete the flight once to commenced.
- Based on the available evidence, it is very unlikely that the pilot was incapacitated or impaired during the flight.
- There was no evidence of any aircraft system or mechanical anomalies that would have directly influenced the accident. However, as a consequence of extensive aircraft damage, it was not possible to be conclusive about the aircraft’s serviceability.
- The aircraft was fitted with Garmin GNS 430W GPS/navigational units that could be configured to provide visual (but not aural) terrain alerts. However, it could not be determined whether the terrain awareness function was selected on during the accident flight.
Safety issues and actions
Central to the ATSB’s investigation of transport safety matters is the early identification of safety issues. The ATSB expects relevant organisations will address all safety issues an investigation identifies. Depending on the level of risk of a safety issue, the extent of corrective action taken by the relevant organisation(s), or the desirability of directing a broad safety message to the aviation industry, the ATSB may issue a formal safety recommendation or safety advisory notice as part of the final report. All of the directly involved parties were provided with a draft report and invited to provide submissions. As part of that process, each organisation was asked to communicate what safety actions, if any, they had carried out or were planning to carry out in relation to each safety issue relevant to their organisation. Descriptions of each safety issue, and any associated safety recommendations, are detailed below. Click the link to read the full safety issue description, including the issue status and any safety action/s taken. Safety issues and actions are updated on this website when safety issue owners provide further information concerning the implementation of safety action. |
Operator’s risk controls to mitigate CFIT risk
Safety issue number: AO-2020-017-SI-01
Safety issue description: Although the operator had specified a flight profile for a straight-in approaches and stabilised approach criteria in its operations manual, and encouraged the use of stabilised approaches, there were limitations with the design of these procedures. In addition, there were limitations with other risk controls for minimising the risk of controlled flight into terrain (CFIT), including no procedures or guidance for the use of the terrain awareness function on the aircraft’s GNS 430W GPS/navigational units, and limited monitoring of the conduct of line operations.
Regulatory requirements for TAWS
Safety issue number: AO-2020-017-SI-02
Safety issue description: The Australian requirements for installing a terrain avoidance and warning system (TAWS) were less than those of other comparable countries for some types of small aeroplanes conducting air transport operations, and the requirements were not consistent with International Civil Aviation Organization (ICAO) standards and recommended practices. More specifically, although there was a TAWS requirement in Australia for turbine-engine aeroplanes carrying 10 or more passengers under the instrument flight rules:
- There was no requirement for piston-engine aeroplanes to be fitted with a TAWS, even though this was an ICAO standard for such aeroplanes authorised to carry 10 or more passengers, and this standard had been adopted as a requirement in many comparable countries.
- There was no requirement for turbine-engine aeroplanes authorised to carry 6–9 passengers to be fitted with a TAWS, even though this had been an ICAO recommended practice since 2007, and this recommended practice had been adopted as a requirement in many comparable countries.
Regulatory guidance for stabilised approach criteria
Safety issue number: AO-2020-017-SI-03
Safety issue description: Although an applicable height of 1,000 ft for stabilised approach criteria in instrument meteorological conditions has been widely recommended by organisations such as the International Civil Aviation Organization for over 20 years, the Civil Aviation Safety Authority had not provided formal guidance information to Australian operators regarding the content of stabilised approach criteria.
Safety action not associated with an identified safety issue
Whether or not the ATSB identifies safety issues in the course of an investigation, relevant organisations may proactively initiate safety action in order to reduce their safety risk. All of the directly involved parties are invited to provide submissions to this draft report. As part of that process, each organisation is asked to communicate what safety actions, if any, they have carried out to reduce the risk associated with this type of occurrences in the future. The ATSB has so far been advised of the following proactive safety action in response to this occurrence. |
Additional safety action by Aerotrack
The owner of Aerotrack has made a change to the tool to ensure compliance with Civil Aviation Safety Regulation 61.875. As a result, only instrument flight rules (IFR) flights with over 1 hour IFR flight time were included in the calculation of recency, and decimals were not included in the calculation.
Additional safety action regarding instrument approach types
A barometric vertical navigation (Baro-VNAV) approach with vertical guidance (APVs) enables a suitably-equipped aircraft’s systems to compute and display a vertical guidance path. Accordingly, they are a 3D instrument approach. Airservices Australia has been implementing Baro-VNAV APV approaches since 2016. After the accident (in December 2020), a Baro-VNAV approach was implemented at Lockhart River (and several other airports in Australia). However, this type of approach requires the aircraft to have a flight management system and VH-OZO was not equipped to conduct a Baro-VNAV approach.
Additional safety action regarding recurrent proficiency checks
On 2 December 2021, CASR Part 135 (Australian air transport operations – smaller aeroplanes) commenced. Air transport operations included passenger transport operations, regardless of whether they were scheduled or charter operations.
It included a requirement for operators to conduct proficiency checks on pilots, with the requirements for such checks to be specified in the Manual of Standards (MOS). The MOS for Part 135 defined a flight crew member proficiency check as:
… an assessment, conducted by an aeroplane’s operator in accordance with the operator’s exposition, of whether a person is competent to safely carry out the person’s duties as a flight crew member in the aeroplane, which relates to the matters mentioned in subsection 12.05(2).
Standard 12.08 stated:
(3) The flight crew member must successfully undertake the operator’s flight crew member proficiency check, for the relevant type or class of aeroplane, as follows:
(a) for a flight crew member only conducting a flight under the VFR by day — subject to subsections (4) and (5), initially 6 months after first commencing unsupervised line operations for the operator, and then at intervals of 1 year after the previous proficiency check;
(b) otherwise — subject to subsections (4) and (5), initially 6 months after first commencing unsupervised line operations for the operator, and then at intervals of 6 months after the previous proficiency check.
For the 1-year requirement, the check could be done within 90 days of the required date and for the 6-months requirement the check could be done within 30 days of the required date.
The matters specified in 12.05(2) were:
(a) training in the duties and responsibilities for the flight crew member’s position;
(b) training in the standard operating procedures for the type or class of aeroplane used for the flight;
(c) training in the normal, non-normal and emergency procedures for an aeroplane of that type or class;
(d) training in any flight procedures or manoeuvres, conducted in an aeroplane of that type or class, for which the operator holds an approval under regulation 91.045, or 135.020, of CASR;
Note: Examples of approvals issued under regulation 91.045, or 135.020, of CASR include approvals to conduct low visibility operations and flights using certain PBN navigation specifications.
(e) training in the procedures for any other operations conducted by the operator in an aeroplane of that type or class that the flight crew member has not previously experienced, for example, precision runway monitor operations or land and hold short operations.
Glossary
ADS-B Automatic dependent surveillance-broadcast
AFRU Aerodrome frequency response unit
AGL Above ground level
AIP Aviation information publication
AOC Air operator’s certificate
APV Approach with vertical guidance
ATC Air traffic control
AWIS Automated weather information service
AWS Automatic weather station
BARS Basic aviation risk standard (a Flight Safety Foundation program)
BoM Bureau of Meteorology
CASA Civil Aviation Safety Authority
CASR Civil Aviation Safety Regulations
CDI Course deviation indicator
CFIT Controlled flight into terrain
CTAF Common traffic advisory frequency
DETRESFA Distress phase
EFB Electronic flight bag
EST Eastern Standard Time
ETA Estimated time of arrival
FAF Final approach fix
FLTA Forward looking terrain avoidance (a function of the Garmin GNS 430W unit)
FMS Flight management system
FSF Flight Safety Foundation
GAF Graphical area forecast
GNSS Global navigation satellite system
GPS Global positioning system
GPWS Ground proximity warning system
GPWT Grid point wind and temperature chart
HF High frequency
IAF Initial approach fix
ICAO International Civil Aviation Organization
IF Intermediate fix
IFR Instrument flight rules
ILS Instrument landing system
IMC Instrument meteorological conditions
INCERFA Uncertainty phase
IPC Instrument proficiency check
JRCC Joint Rescue Coordination Centre
LCD Liquid crystal display
LNAV Lateral navigation
MAPt Missed approach point
MDA Minimum descent altitude
METAR Meteorological aerodrome report
MOPSC Maximum operational passenger seat configuration
NAIPS National Aeronautical information Processing System
NM Nautical miles
NOTAM Notice to airmen
NPRM Notice of proposed rule making
OPC Operator proficiency check
PDA Premature descent alert (a function of the Garmin GNS 430W unit)
PLB Personal locator beacon
POH Pilot’s operating handbook
QNH That pressure setting which, when placed on the pressure setting sub‑scale of a sensitive altimeter of an aircraft located at the reference point of an aerodrome, will cause the altimeter to indicate the vertical displacement of the reference point above mean sea level.
RAIM Receiver autonomous integrity monitoring
RNAV Area navigation
RNP Required navigation performance
SIGMET Significant meteorological information
SMS Safety management system
SPFIB Specific pre-flight information bulletin
TAF Aerodrome forecast
TAWS Terrain avoidance and warning system
VFR Visual flight rules
VHF Very high frequency
VMC Visual meteorological conditions
VNAV Vertical navigation
Vref Reference landing speed
VSI Vertical speed indicator
Sources and submissions
Sources of information
The sources of information during the investigation included the:
- operator/chief pilot of Air Connect Australia
- aircraft owner and maintainer for VH-OZO
- GPS unit manufacturer (Garmin)
- Civil Aviation Safety Authority
- Queensland Police Service
- Airservices Australia
- Bureau of Meteorology
- OzRunways flight data.
References
Arthur W, Bennett W, Stanush PL and McNelly TL (1998) ‘Factors that influence skill decay and retention: A quantitative review and analysis’, Human Performance, 11:57-101.
Australian Transport Safety Bureau (2007) CFIT: Australia in context 1996 to 2005.
Civil Aviation Authority (2013) Monitoring matters: guidance on the development of pilot monitoring skills, CAA Paper 2013/02.
Dismukes RK, Berman BA & Loukopoulos LD (2007) The limits of expertise: Rethinking pilot error and the causes of airline accidents, Ashgate, Aldershot UK.
Enders JH, Dodd R, Tarrel R, Khatwa R, Roelen ALC & Karwal AK (1996) Airport safety: a study of accident and available approach-and-landing aids, Flight Safety Foundation, Flight Safety Digest, 15(3).
European Union Agency Safety Agency (2021), Safety issue report – Skills and knowledge degradation due to lack of recent practice, downloaded from www.easa.europa.eu.
Federal Aviation Administration (2012) Instrument flying handbook, FAA-H-8083-15B
Flight Safety Foundation 2000a ‘ALAR briefing note 7.1 – Stabilized approach’, Flight Safety Digest, August-November 2000.
Flight Safety Foundation 2000b ‘ALAR briefing note 5.3 – Visual illusions’, Flight Safety Digest, August-November 2000.
Gibbs R, Gray R & Scharff L (2010) Aviation visual perception: Research, misperception and mishaps, Ashgate, Aldershot UK.
Godley ST (2006) Perceived pilot workload and perceived safety of RNAV (GNSS) approaches, Australian Transport Safety Bureau.
Hoekstra HD, Perry EB & Huang S (1972) Altimetry display study Part 2 – Analysis of altitude accidents, Report No. FAA-RD-72-46 II, prepared by the Flight Safety Foundation for the US Department of Transportation, Federal Aviation Administration.
Holmes S, Bunting A, Brown D, Hiatt K, Braithwaite M & Harrigan M (2003) ‘Survey of spatial disorientation in military pilots and navigators’, Aviation, Space, and Environmental Medicine, 74:957-965.
International Air Transport Association (IATA) (2018) Controlled flight into terrain accident analysis report 2008–2017 data, Montreal, Canada
Kelly D & Efthymiou M (2019) ‘An analysis of human factors in fifty controlled flight into terrain aviation accidents from 2007 to 2017’, Journal of Safety Research, 69:155-165.
Michalski DJ & Bearman C (2014) ‘Factors affecting the decision making of pilots who fly in Outback Australia’, Safety Science, 68:288-293.
Mitchell TR (1972) Altimetry display study Part 1 – Summary report, Report No. FAA-RD-72-46 I, prepared by The Mitre Corporation for the US Department of Transportation, Federal Aviation Administration.
Orlady HW & Orlady LM (1999) Human factors in multi crew operations, Ashgate, Aldershot, UK.
Paletz SBF, Bearman C, Orasanau J & Holbrook J (2009) ‘Socializing the human factors analysis and classification system: Incorporating social psychological phenomena into a human factors error classification system’, Human Factors, 51:435-445.
Previc FH (2004) ‘Visual illusions in flight’, in FH Previc & R Ercoline (Eds) Spatial disorientation in aviation, American Institute of Aeronautics and Astronautics, Reston VA.
Sanli EA and Carnahan H (2018) ‘Long-term retention of skills in multi-day training contexts: A review of the literature’, Industrial Journal of Ergonomics, 66:10–17.
Shrager JJ (1972) Altimetry display study Part 3 – Review of R&D on display readability, Report No. FAA-RD-72-46 III, prepared by the Navigational Aviation Facilities Experimental Center for the US Department of Transportation, Federal Aviation Administration.
Staal MA (2004) Stress, cognition, and human performance: A literature review and conceptual framework, National Aeronautics and Space Administration Technical Memorandum NASA/TM-2004-212824.
Vlasblom JID, Pennings HJM, Van der Pal J and Oprins EAPB (2020) ‘Competence retention in safety-critical professions: A systematic literature review’, Educational Research Review, 30:10.1016.
Wickens CD, Hollands JG, Banbury S & Parasuraman R (2013) Engineering psychology and human performance, 4th edition, Pearson Boston, MA.
Submissions
Under section 26 of the Transport Safety Investigation Act 2003, the ATSB may provide a draft report, on a confidential basis, to any person whom the ATSB considers appropriate. That section allows a person receiving a draft report to make submissions to the ATSB about the draft report.
A draft of this report was provided to the following directly involved parties:
- the aircraft operator
- the aircraft owner/maintainer
- the Civil Aviation Safety Authority
- Airservices Australia
- Bureau of Meteorology
- Aerotrack
- United States National Transportation Safety Board
- the aircraft manufacturer
- Garmin.
Submissions were received from:
- the aircraft operator
- the Civil Aviation Safety Authority
- Bureau of Meteorology
- Garmin.
The submissions were reviewed and, where considered appropriate, the text of the report was amended accordingly.
Appendices
Appendix A – Research and guidance regarding design of altimeters
Civil Aviation Order (CAO) 20.18 (Aircraft equipment — basic operational requirements) required that aeroplanes engaged in passenger charter operations under the instrument flight rules (IFR) be equipped with 2 sensitive pressure altimeters. As already noted in Aircraft instruments, VH‑OZO was fitted with two 3-pointer altimeters that met this requirement.
A series of research studies have compared the effectiveness different types of altimeter display. In summarising the research from 1960 to 1972 for the United States Federal Aviation Administration (FAA), Shrager (1972) concluded:
3. Comparative laboratory, simulator, and flight tests of 3P [3-pointer], DP [drum-pointer], CP counter-pointer], and CDP [counter drum pointer] altimeter displays[76] indicate that the least preferred was the 3P and the most desirable was the CDP by subject opinion. This order of ranking is most pronounced when the subjects used were pilots as opposed to nonpilots. The subjective display preference was further accentuated when random dynamic changes in altitude and secondary tasks were introduced into the experiment.
4. The 3P display required the longest time to interpret and produced the largest number of errors among all the production-type displays evaluated. The misreading errors included both those of l,000- and 10,000-foot altitudes. The experimental test results have been supported by significant actual flight experiences on the part of both the military and commercial aviation.[77]
5. A majority of the air carriers and the military have or are converting to displays other than the 3P. The principal display selected has been the CDP.
6. The most prevalent type of altimeter display in general aviation is the 3P. The 10,000-foot error of the 3P display has not been supported by significant actual flight experience by general aviation. However, the 1,000-foot error is a problem in the 3P display.
As part of a related research program, Hoekstra and others (1972) reviewed civil and military accidents during 1964–1970. They noted that problems with misreading an altimeter were cited in a small number of accident investigation reports. For the examples provided, where the type of altimeter was stated or otherwise known, the majority involved 3-pointer altimeters. This study also conducted a survey of various organisations. Its conclusions included:
1. Official investigations have cited altimetry as a causal factor in approximately 1% of all accidents; considering accidents of unknown causes and those where altimetry could possibly have been a factor, it is estimated that altimetry contributes to a maximum of 3% of all accidents. This estimate includes mechanical malfunctions and human errors.
2. Ten to twenty percent of all accidents are "altitude-related" as they involve altitude displacement for some reason.
3. "Altitude-related" accidents occur more frequently in conditions where it is difficult to see the terrain, i.e., at night and in restricted visibility.
4. The majority of "altitude-related" accidents occur during the landing approach. Most are without benefit of vertical guidance from any source other than the altimeter and pilot visual contact with the terrain.
5. Although a few accidents occurred in landing approaches where precision aids were used, they were characterized by a deviation from the glide path after the pilot gained visual contact.
6. Survey comments indicated that the three-pointer altimeter is susceptible to frequent misreading by 1,000 or 10,000 feet, but the number of accidents known to have been caused or possibly could have been caused, by this type of misreading was small. However, several incidents of misreading were reported.
7. Small improvements in the accident rate could be achieved by improving altimetry display, static/pitot systems and setting procedures, but greater improvement would result from the provision of more precision aids and visual warning systems in the ground environment.
A report summarising the FAA research program (Mitchell 1972) concluded that retrofitting of aircraft was not justified. However, it recommended that an advisory circular should be distributed, suggesting that all 3-pointer and drum-pointer altimeters be replaced with counter-drum pointer altimeters where feasible, and that counter-drum pointer altimeters should be required for all yet-to-be-constructed transport category aircraft and more expensive general aviation aircraft.
In 1991, the Flight Safety Foundation launched a campaign to reduce the number of controlled flight into terrain (CFIT) accidents and, together with other organisations, it formed a task force. As part of its work, the FSF CFIT task force made 8 recommendations to the International Civil Aviation Organization (ICAO), including a warning against the use of 3-pointer and drum-pointer altimeters.
ICAO Annex 6 (Operation of Aircraft) Part I (International Commercial Air Transport – Aeroplanes) paragraph 6.9.1 required that aeroplanes operated in accordance with IFR (for commercial air transport) be equipped with 2 sensitive pressure altimeters. Since 1998, it also required that these altimeters have ‘counter drum-pointer or equivalent presentation’. A note under this requirement stated that 3-pointer or drum-pointer altimeters did not satisfy this requirement.[78]
All modern transport aircraft are equipped with vertical altimeter displays or, for some older transport aircraft, counter-drum pointer altimeters. However, 3-pointer altimeters are still very common in general aviation aircraft, including small aeroplanes used for passenger transport activities.
Several experienced pilots interviewed by the ATSB during the investigation reported that misreading a 3-pointer altimeter is more commonly seen in pilots undergoing initial instrument training.
Appendix B – Vacuum system analysis
System overview
VH-OZO had 2 artificial horizon (attitude indicator) instruments and one directional gyro instrument that were powered through hoses connected to a common manifold with 2 sources of power from a vacuum pump on each engine, controlled by in-line regulators. An analogue suction indicator (vacuum gauge) located on the lower left pilot-side instrument panel showed the pilot the net amount of suction at the manifold and ‘dolls eye’ indicators showed if each vacuum pump was operating or not.
Maintenance history
Both artificial horizon instruments and directional gyro instrument as well as vacuum regulators were not subject to service life or maintenance requirements, other than regulator garter filter changes that were being carried out. According to a service bulletin issued by the manufacturer of the vacuum pumps, the vanes could be inspected through a wear indicator port and it recommended the pump be replaced at the wear limit.
The left vacuum pump had been in service for about 1,076 hours and the right for about 911 hours, which exceeded the life of the previous pumps (711 hours and 880 hours respectively). The previous pumps were replaced due to failing in service rather than due to wear. Inspection of pump wear through a port was not carried out consistently by the aircraft maintainer and it is unlikely it was conducted at the previous periodic inspection.
The ATSB identified that the vacuum system manifold had not been tested in the previous 5 years or replaced in the previous 10 years, as specified by the component manufacturer. This was due to an oversight in the maintenance tracking system that was subsequently rectified. A search of the aircraft logbooks did not identify any recorded replacement of the manifold and markings that might indicate component life were indistinct.
Pilots and engineers can carry out an informal test of the manifold by alternating the engine that is started (and/or shut down) first and verifying that either vacuum pump by itself can maintain adequate supply. This was not noted at the recent 100-hour inspection and it is not known if the pilot routinely monitored the operation of each vacuum pump at engine start or shutdown. Damage sustained in the accident prevented functional testing and examination of the internal condition of the manifold.
Although the vacuum manifold was outside its specified service life and its condition could not be verified, if there was an age-related defect there was no effect on aircraft operation unless one of the vacuum pumps failed.
Post-accident inspection
Both vacuum pumps were recovered from the aircraft after the accident and examined.
The right vacuum pump showed evidence of casing deformation and damage consistent with a heavy impact sustained during the accident sequence. When the right vacuum pump was removed from the engine the drive shaft was observed to have sheared. An accurate wear measurement could not be ascertained due to internal damage within the unit. Inspection of the internal components indicated that the pump vanes were intact, and the carbon block had fractured. The support pin had also failed in overload. Inspection of the frangible drive shaft showed damage indicative of overload. Further examination of the frangible shaft fracture surface under a microscope did not identify any features indicative of surface-to-surface rotation contact that may be possible when the engine is running and the pump is seized. Post-accident examination concluded that it was highly likely the pump failed due to the impact with terrain.
The left vacuum pump showed no sign of external damage and the drive shaft was intact. The pump did not show any signs of accident damage. An accurate wear measurement could not be conducted because the pump did not have an inspection port. Inspection of the internal components indicated that the pump vanes and carbon block were intact. The pump internal components were rotated and observed to work as designed. There were no pre- or post-impact defects identified within the left vacuum pump that would make it incapable of supplying pressure as required.
The left artificial horizon instrument and directional guidance instrument were recovered from the aircraft after the accident, disassembled and examined. The left artificial horizon instrument was significantly damaged, however detailed examination of the components did not identify any pre-impact defects. Rotational scoring was noted between the rotor, the casing and the end cap with metal transfer evident (Figure 22). This indicates that the rotor was rotating with significant speed, highly likely produced by suction from the vacuum pump(s), when the aircraft impacted with terrain.
Figure 22: Left artificial horizon internal components showing rotational contact damage
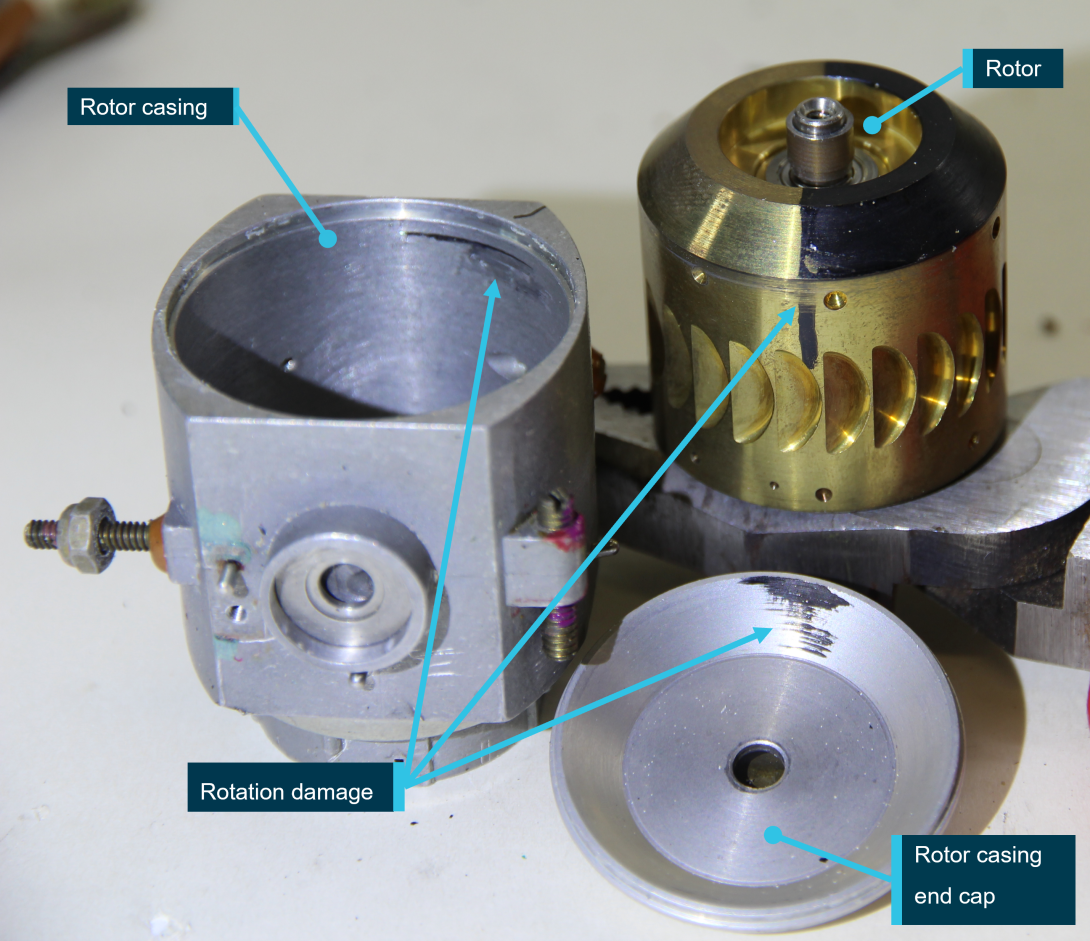
Source: ATSB
The directional gyro instrument was significantly damaged, with detailed examination producing no identified pre-impact defects with the components that were available for inspection. There was evidence of rotational scoring on the rotor case end and the rotor end. There was no scoring evidence in the rotor case. The rotational scoring indicates that the directional gyro was rotating at significant speed, highly likely produced by suction from the vacuum pump(s), when the aircraft impacted with terrain.
Appendix C – Detailed 1-minute weather data Lockhart River 0850–0930
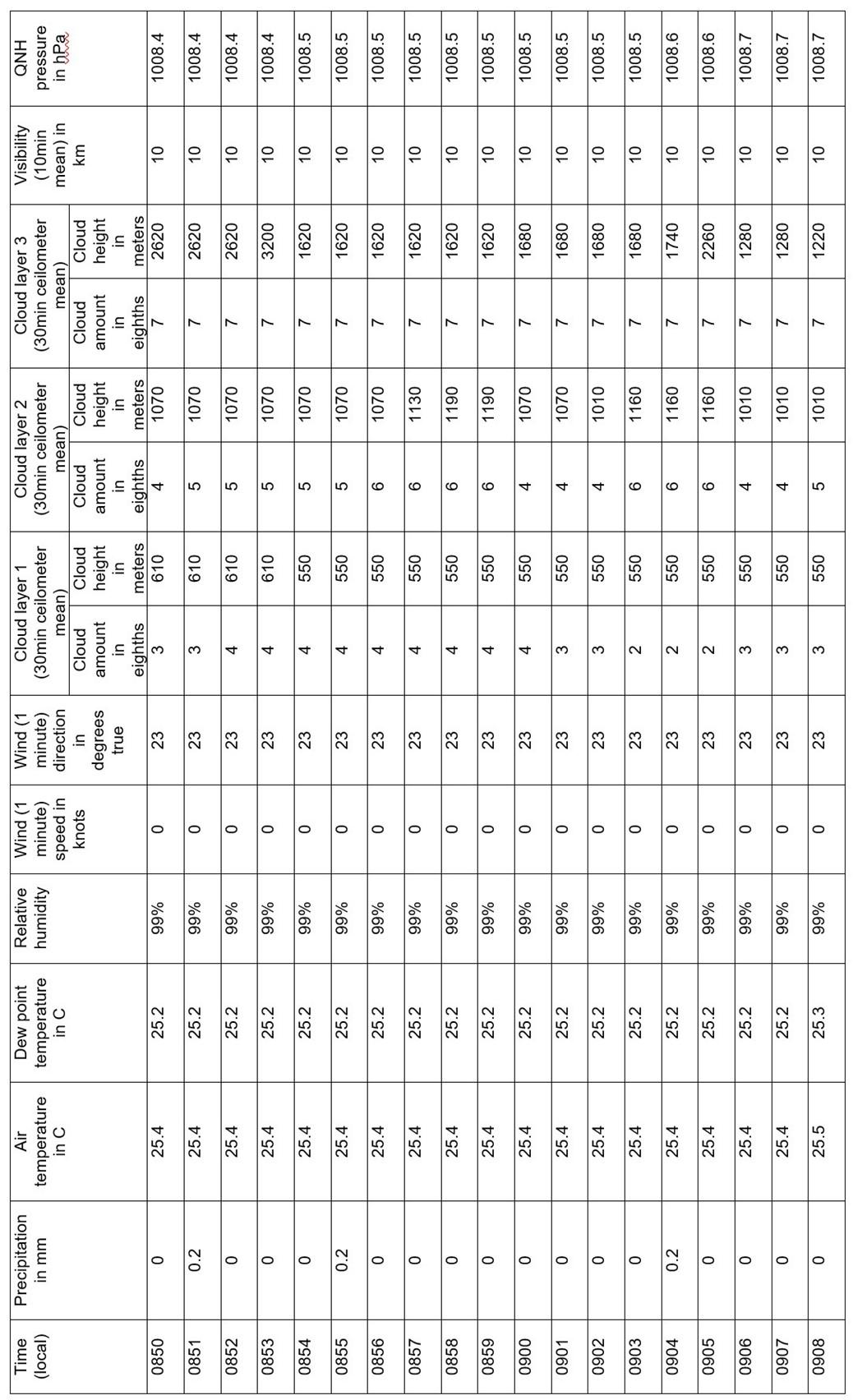
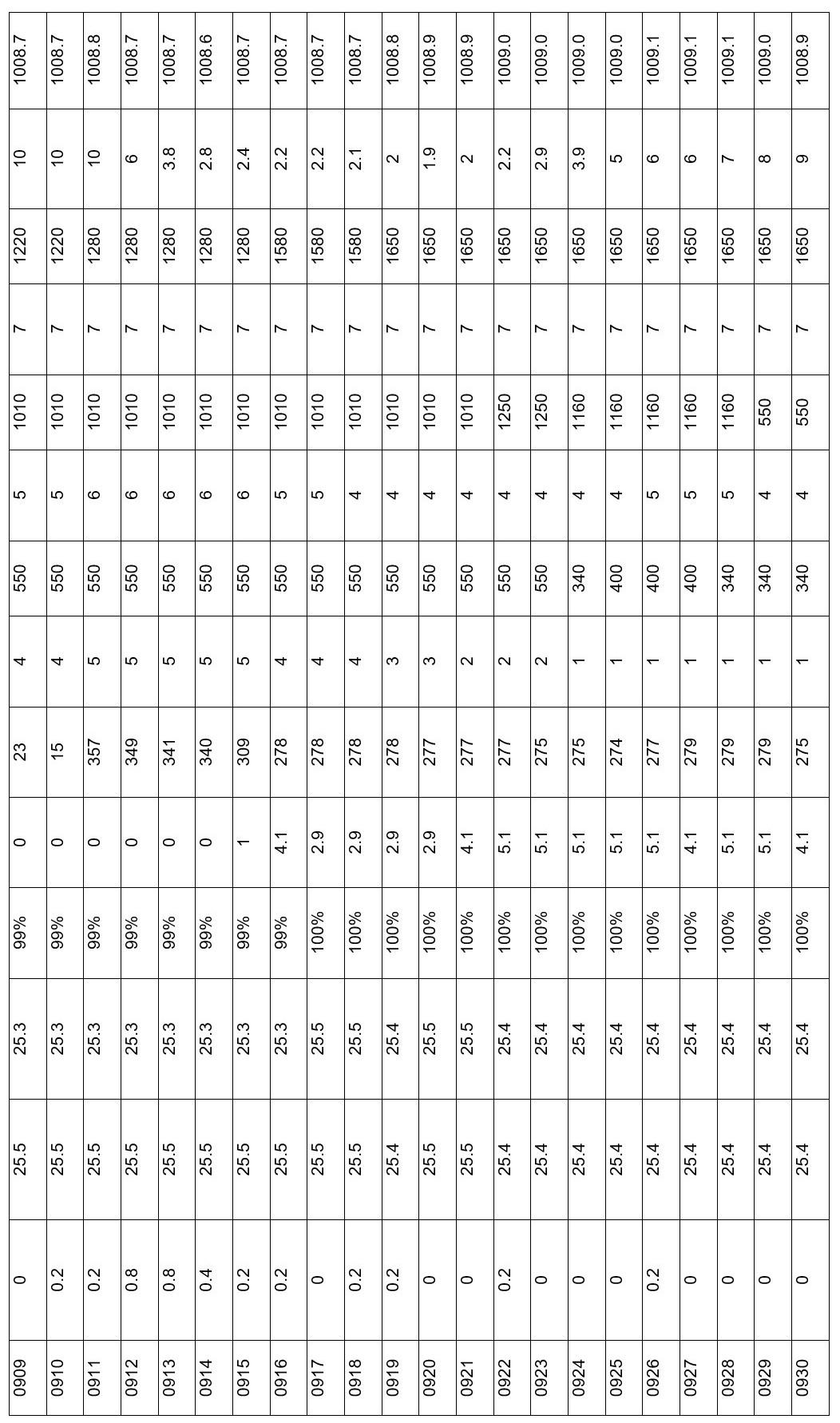
Appendix D – Guidance to industry regarding stabilised approaches
Overview
This appendix provides examples of guidance provided regarding stabilised approach criteria for flight operations in addition to that provided by the Flight Safety Foundation.
Transport Canada guidance
Transport Canada’s Advisory Circular AC700-028 (Vertical path control on non-precision approaches, issued 2013) was applicable to all flight crew and types of operations. It was issued in 2013, at the same time that NAV CANADA introduced non-precision approach (NPA) charts with constant descent angles. In terms of stabilised approaches, the AC stated:
Many air operators require their crews to use a stabilized approach technique which is entirely different from that envisaged in the original NPA procedure design. A stabilized approach is calculated to achieve a constant rate of descent at an approximate 3° flight path angle; with stable airspeed, power setting, and attitude; and with the aircraft configured for landing. The safety benefits derived from a stabilized final approach have been recognized by many organizations including ICAO, the Federal Aviation Administration, and Transport Canada Civil Aviation (TCCA). Those air operators not already doing so are encouraged to incorporate stabilized approach criteria into their Standard Operating Procedures (SOPs) and training syllabi.
With regard to reference points for stabilised approach criteria, the AC stated:
Stabilized approach criteria should be defined for all approaches and may include:
-
- that flights shall be stabilized by no lower than 1,000 feet (ft.) above the threshold when in instrument meteorological conditions (IMC);
- that all flights shall be stabilized by no lower than 500 ft. above the threshold;
- that the flight remain stabilized until landing
- that if an approach is not stabilized in accordance with these requirements, or has become destabilized afterwards, a go-around is required.[79]
The AC also provided detailed comments regarding step-down versus constant descent angle approach techniques. It noted that step-down techniques were inherently unstable and resulted in higher workload. They also resulted in inconsistent rates of descent and at times high rates of descent, and also extended periods with the aircraft flown at minimum safe altitudes. In contrast, constant descent angle approaches were inherently stable and associated with lighter workload.
In 2015, Transport Canada also issued a Civil Aviation Safety Alert 2015-04 (Stabilized approach) for all commercial air operators (including airline, commuter, air taxi and aerial work). It reiterated the key points of the AC, and noted that:
Rushed and unstabilized approaches remain a significant factor in Controlled Flight Into Terrain (CFIT) and other Approach-and-Landing Accidents (ALA). The safety benefits derived from a stabilized final approach have been recognized by many organizations including ICAO, the FAA, EASA and TCCA. These benefits include:
- Increased flight crew situational awareness;
- More time and attention for monitoring ATC communications, weather conditions and systems operation;
- More time and attention for flight path and energy monitoring;
- Defined flight parameter deviation limits and minimum stabilization heights to support the decision to land or to go-around; and,
- Landing performance consistent with expected performance values.
It also stated:
Stabilized approach criteria should be defined for all approaches and should include that:
- Approaches be stabilized by no lower than 1,000 feet (ft) above aerodrome elevation (AAE) when in instrument meteorological conditions (IMC);
- All approaches be stabilized by no lower than 500 ft AAE in visual meteorological conditions (VMC);
- A call be made upon reaching 1000 ft AAE in IMC or 500 ft AAE in VMC as to whether the approach is stabilized or not;
- The approach remain stabilized until landing;
- If an approach is not stabilized in accordance with these requirements, or has become destabilized afterwards, a go-around is required.
Federal Aviation Administration guidance
The United States Federal Aviation Administration issued guidance for general aviation pilots regarding CFIT in Advisory Circular AC 61-134 (General aviation controlled flight into terrain awareness), issued in 2003. The background section stated:
According to FAA information, general aviation CFIT accidents account for 17 percent of all general aviation fatalities. More than half of these CFIT accidents occurred during IMC…
Because a single-piloted, small GA aircraft is vulnerable to the same CFIT risks as a crewed aircraft but with only one pilot to perform all of the flight and decision-making duties, that pilot must be better prepared to avoid a CFIT type accident…
Under a section regarding GA operations in IMC on an IFR flight, the AC stated:
These operations also pose special risks. Whether it is failure to follow safe takeoff and departure techniques, recommended en route procedures ― which includes loss of situational awareness ― or failure to maneuver safely to a landing, IFR operations can be dangerous for those not prepared to operate or not current and proficient in the IMC and IFR environments. Many of these accidents result in fatalities. Techniques or suggestions for avoiding some of these IFR risk factors include…
s. The importance of flying a stabilized approach. A common definition of a stabilized approach is maintaining a stable speed, descent rate, vertical flightpath, and configuration throughout the final segment of the approach. Although originally designed for turbojet aircraft, a stabilized approach is also recommended for propeller-driven aircraft. The idea is to reduce pilot workload and aircraft configuration changes during the critical final approach segment of an approach. The goal is to have the aircraft in the proper landing configuration, at the proper approach speed, and on the proper flightpath before descending below the minimum stabilized approach height. The following are recommended minimum stabilized approach heights.
(1) 500 feet above the airport elevation during VFR weather conditions.
(2) MDA or 500 feet above airport elevation, whichever is lower, for a circling approach.
(3) 1,000 feet above the airport or touch down zone elevation during IMC.
In 2011, the United States Federal Aviation Administration (FAA) issued AC 120-108 (Continuous descent final approach), providing guidance for operators on the continuous descent final approach technique for NPAs. The AC was intended for airline and air taxi operators, though it noted the guidance was beneficial to all operators. The background section stated:
Controlled flight into terrain (CFIT) is a primary cause of worldwide commercial aviation fatal accidents. Unstabilized approaches are a key contributor to CFIT events. Present NPAs are designed with and without stepdown fixes in the final approach segment. Stepdowns flown without a constant descent will require multiple thrust, pitch, and altitude adjustments inside the final approach fix (FAF). These adjustments increase pilot workload and potential errors during a critical phase of flight. NPAs designed without stepdown fixes in the final segment allow pilots to immediately descend to the MDA after crossing the FAF. In both cases, the aircraft remains at the MDA until descending for the runway or reaching the missed approach point (MAP). This practice, commonly referred to as “dive and drive,” can result in extended level flight as low as 250 feet above the ground in instrument meteorological conditions (IMC) and shallow or steep final approaches.
In terms of stabilised approaches, the AC 120-08 stated:
A stabilized approach is a key feature to a safe approach and landing. Operators are encouraged by the FAA and the International Civil Aviation Organization (ICAO) to use the stabilized approach concept to help eliminate CFIT. The stabilized approach concept is characterized by maintaining a stable approach speed, descent rate, vertical flightpath, and configuration to the landing touchdown point. Depart the FAF configured for landing and on the proper approach speed, power setting, and flightpath before descending below the minimum stabilized approach height; e.g., 1,000 feet above the airport elevation and at a rate of descent no greater than 1,000 feet per minute (fpm), unless specifically briefed. (Refer to AC120-71.)
The FAA also issued guidance regarding stabilised approaches in AC 91-79A (Mitigating the risks of a runway overrun upon landing), issued in 2014 and last updated in 2018. The intended audience was all pilots, flight crew and operators. The AC stated in part:
Stabilized on Profile. The airplane should be stabilized on profile before descending through the 1,000-ft window or through the 500 ft above touchdown zone elevation (TDZE) window in visual meteorological conditions (VMC). Configuration, trim, speed, and glidepath should be at or near the optimum parameters early in the approach to avoid distractions and conflicts as the airplane nears the threshold window. The electronic or visual glidepath or an optimum glidepath angle of 3 degrees should be established and maintained…
Indicated Airspeed. Indicated airspeed should be not more than VREF + 5 or the POH published approach airspeed, with appropriate adjustments for wind or other factors, and never less than VREF or the appropriate airspeed in order to avoid the loss of aircraft control.
United States Aircraft Owners and Pilots Association
The United States Aircraft Owners and Pilots Association (AOPA) stated in a 2000 article (The stabilized approach):
Most of us don’t have to worry about the vagaries of turbine aircraft and their response to power and speed changes on approach. But there are plenty of good reasons to fly stabilized approaches regardless of whether you fly a Cessna Skyhawk or Golden Eagle, Beech King Air or Piper Super Cub. Here are a few:
- It reduces workload…
- It gives us more opportunity to see the big picture…
- It slows the aircraft down earlier, allowing more time to think…
- It allows more time to react...
- It makes it easier to fly the VOR, ILS, or ADF needles...
- It allows the pilot more opportunity to detect changes in the wind on approach…
- It reduces the variables and thus reduces our required reactions to these changes. When you have the airspeed nailed early, you have one less variable to worry about, freeing you to focus on other things. If we can set the power and mostly forget it, we don't have to constantly change power in response to our configuration and speed changes.
- It creates the time to finish your before-landing checklist and to really look around the airplane for other things you might have missed or neglected when rushed...
- It makes the approach—and thus the landing spot—predictable because you do it the same every time.
Notice that time is the major benefit when we fly a stabilized approach. Time allows us to more easily perceive changes and then make corrections to course, altitude, or airplane management.
Most airlines set the stabilized approach point at 500 feet in visual conditions and 1,000 feet when the weather is IMC. These 500- and 1,000-foot target points might be a good starting place when establishing your own stabilized approach minimums. You might also want to use 1,000 feet for night approaches and landings because the fewer visual cues at night reduce your ability to perceive differences and changes.
Appendix E – Aurukun incident flight – 22 January 2020
Introduction
On 22 January 2020, on a flight from Weipa to Aurukun, the pilot conducted 2 RNAV (GNSS) approaches to runway 34 at Aurukun in VH-OZO, with the first approach followed by a missed approach.
An extract of the relevant Airservices Australia approach chart is depicted in Figure 23. As indicated in the chart, the recommended initial approach altitude was 1,800 ft, with the recommended 3° approach profile to the runway threshold commencing just prior to the final approach fix (FAF). The minimum descent altitude (MDA) for a pilot with an actual QNH was 450 ft.
The ATSB analysed OzRunways GPS data from the 2 approaches and the missed approach (Figure 24). The data was recorded at 5-second intervals. Recorded altitude, lateral position and groundspeed data for the 2 approaches from the initial approach fix (IAF) to the missed approach point (MAPt) is shown in Figure 25.
The recorded altitudes were slightly below the recommended approach profile during the 2 approaches. Aurukun Airport has an elevation of about 30 ft above sea level, and one of the passengers recalled observing the altimeter reading 50–100 ft when the aircraft was on the ground after landing. This indicated the QNH set on the altimeter may not have accurately reflected the actual QNH when the aircraft landed,[80] and the altimeter may have been slightly overreading during the approaches. In addition, the recorded altitude data was truncated to the nearest 100 ft.
Figure 23: Extract of the Aurukun RNAV (GNSS) RWY 34 approach
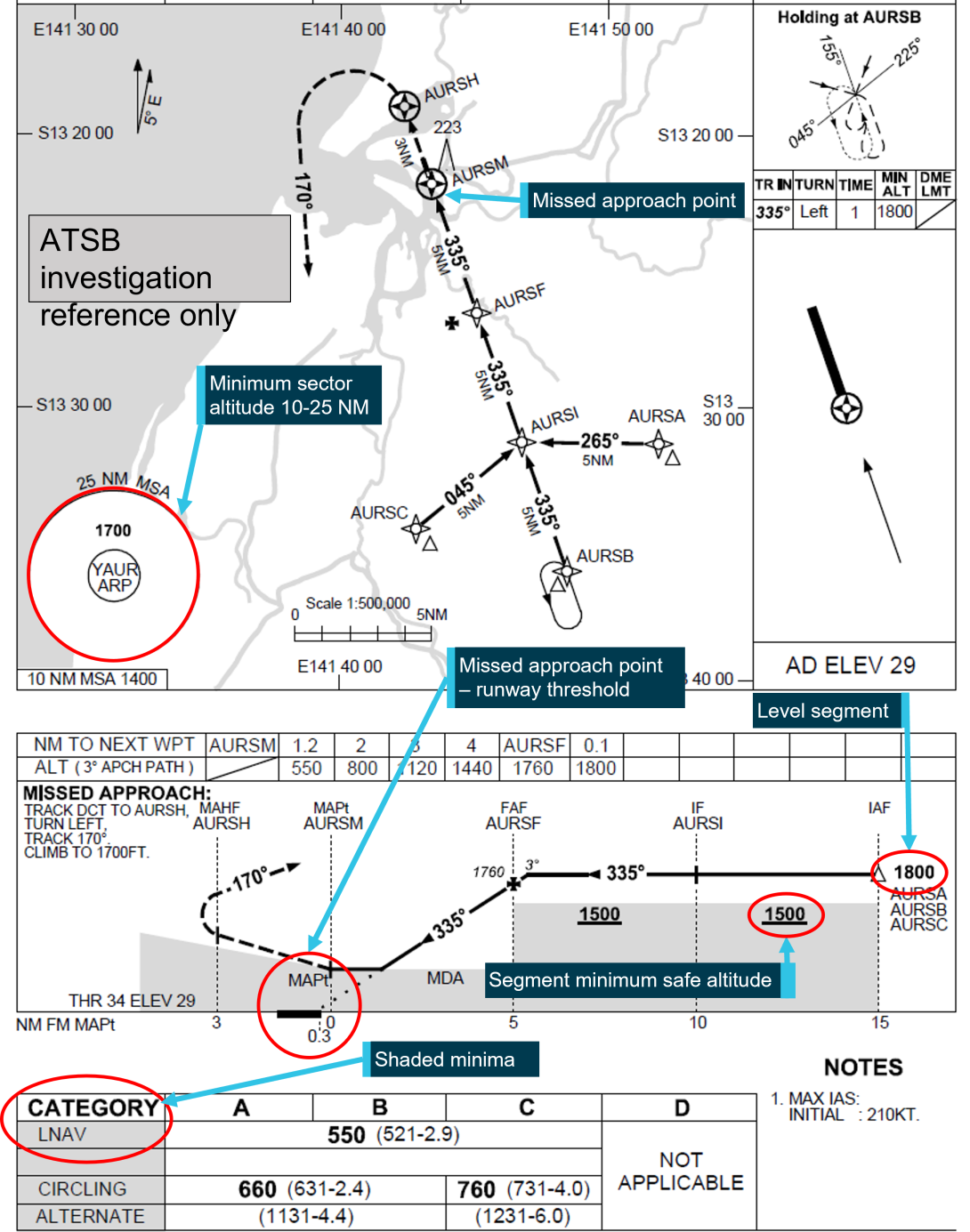
Source: Airservices Australia, annotated by the ATSB
Figure 24: VH-OZO recorded data 22 January 2020 at Aurukun, Queensland
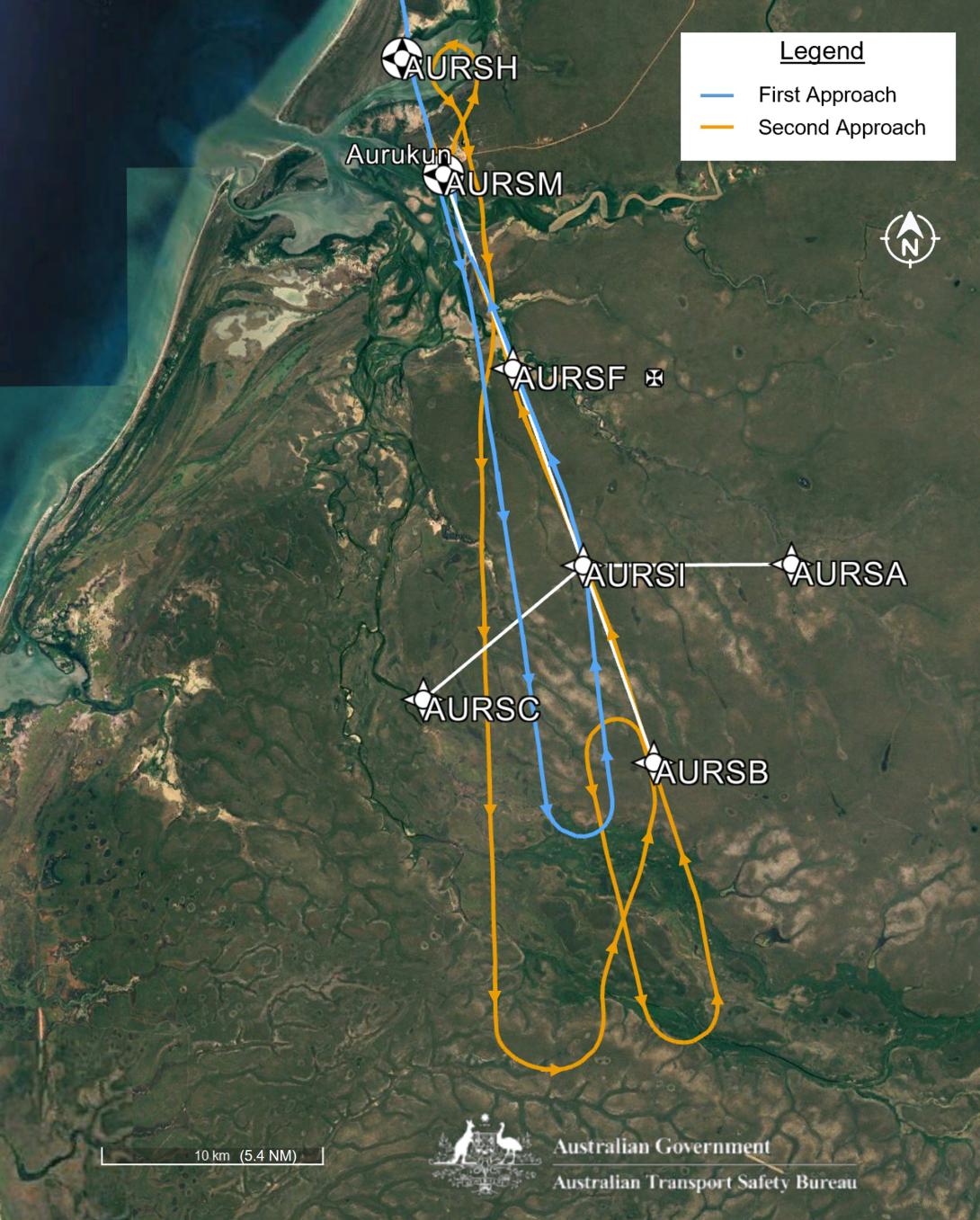
Source: Google Earth overlaid with OzRunways data, annotated by the ATSB
First approach
The data showed that the aircraft approached from the north and about 1,100 ft and overflew the runway 34 threshold before continuing to track south. After passing about 3 NM west of the initial approach fix (IAF) AURSB at 1,500 ft, the aircraft turned 180° then passed 1 NM to the west of the IAF and then tracked direct towards the intermediate fix (IF) AURSI at 1,500 ft. About 1 NM prior to AURSI, the aircraft climbed to 1,700 ft and passed over the IF.
The aircraft was at 1,600 ft when it reached the FAF AURSF, and it then commenced the descent on about a 3° profile (Figure 25). The aircraft was about 200–300 ft below the recommended 3° descent profile when it reached the MDA, about 1.8 NM prior to the MAPt AURSM. The minimum recorded altitude the aircraft descended to (1 data point) was 200 ft when 1.2 NM prior to the MAPt, and it was at a recorded altitude of 200–300 ft over 4 data points (15–20 seconds) prior to reaching the MAPt.
Figure 25: Recorded data for 2 RNAV approaches to Aurukun 22 January 2020
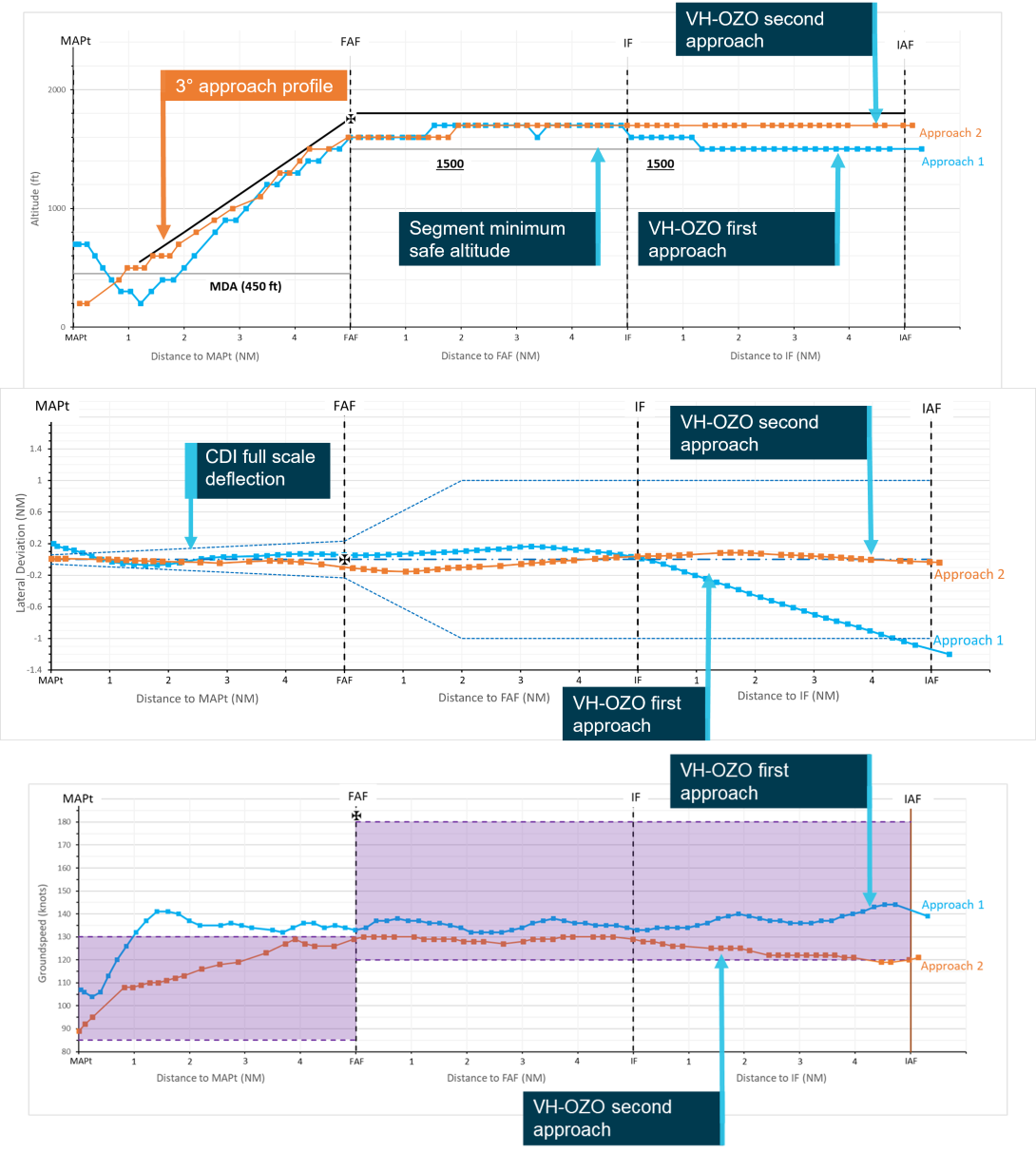
Source: ATSB
The descent rate from the FAF (recorded height 1,600 ft) until the aircraft reached 900 ft was about 650 ft/min, and the descent rate for the remainder of the descent was about 1,050 ft/min.
A review of wind and temperature forecast and analysis charts from multiple sources and other information indicated that the indicated airspeeds would have been about 5 kt higher than the recorded groundspeeds during the 2 approaches while the aircraft was on or near the intermediate/final approach track. Therefore, on the first approach the indicated airspeed was about 140 kt when the aircraft passed the FAF, about 145 kt when the aircraft passed through the MDA and about 140 kt when the aircraft reached a height of 300 ft above aerodrome elevation.
Missed approach
A missed approach was commenced prior to the MAPt, with the aircraft climbing and passing 0.2 NM to the right of the MAPt. The aircraft passed over the Aurukun township at an altitude of about 800 ft, then turned left and heading south, climbing to 1,900 ft.
The published missed approach commenced at AURSM and required the aircraft to maintain runway direction for 3 NM before turning left to heading 170° and climbing to 1,700 ft. Based on witness reports, it is possible that the pilot was avoiding a nearby thunderstorm during the missed approach and/or was in visual conditions at the time.
The exact reasons for the missed approach could not be determined (that is, whether it was due only to reduced visibility of the runway at the time or also due to the aircraft’s speed not meeting the operator’s stabilised approach criteria at 300 ft above aerodrome elevation).
Second approach
The aircraft then headed south to about 8 NM south-south west of AURSB, before turning back to the IAF and then doing a holding pattern before again approaching the IAF. The pilot then commenced a second RNAV approach about 31 minutes after the first approach from an altitude of about 1,900 ft. The aircraft was close to the published descent profile throughout the approach and also within the required lateral tolerances.
Given the indicated airspeeds were about 5 kt higher than the recorded groundspeeds, the indicated airspeeds were 135 kt at the FAF and 105 kt at 300 ft above aerodrome elevation. Overall, the approach appeared to comply with the operator’s stabilised approach criteria.
Appendix F – Related occurrences
Collision with Terrain involving Fairchild Metro 23 aircraft, VH-TFU, 11 km north-west of Lockhart River Airport, Queensland, on 7 May 2005
On 7 May 2005, a Fairchild Aircraft Inc. SA227-DC Metro 23 turbo-prop aircraft, registered VH-TFU, with 2 pilots and 13 passengers, was being operated on an instrument flight rules (IFR) regular public transport service from Bamaga to Cairns, with an intermediate stop at Lockhart River, Queensland. At 1143:39 local time, the aircraft impacted terrain 11 km north-west of the Lockhart River Airport. At the time of the accident, the crew was conducting an area navigation global navigation satellite system (RNAV GNSS) non-precision approach to runway 12. The occupants were fatally injured and the aircraft was destroyed.
The accident was almost certainly the result of a controlled flight into terrain. Weather conditions in the Lockhart River area were poor and necessitated the conduct of an instrument approach procedure for an intended landing at the aerodrome. The cloud base was probably between 500 ft and 1,000 ft above mean sea level and the terrain to the west of the aerodrome, beneath the runway 12 RNAV GNSS approach, was probably obscured by cloud.
The investigated identified a significant number of contributing factors and other safety factors associated with the crew’s performance, local conditions, the operator’s procedures and regulatory oversight. With relevance to the findings of the 2020 accident involving VH-OZO, these included the aircraft descending below the segment minimum safe altitude for the aircraft’s position on the approach (after passing the final approach fix) and the speeds and descent rate exceeding those appropriate for a stabilised approach. The operator’s procedures did not provide clear guidance on approach speeds, when to select aircraft configuration changes during an approach and clear criteria for a stabilised approach. There were also problems with the operator’s processes for supervising the standard of flight operations, and the operator did not have a structured process for managing safety-related risks.
The aircraft was fitted with a ground proximity warning system (GPWS), but there would have been insufficient time for the crew to effectively respond to the GPWS alert and warnings that were probably annunciated during the final 5 seconds prior to impact with terrain. The aircraft was not fitted with a terrain avoidance and warning system (TAWS).
The GPS unit fitted to the aircraft (Garmin GPS 155XL) also had several limitations compared with more recent models available at the time. These included the limited usefulness of the moving map display because of the vertical size of the screen size, the lack of an option to display a distance to the missed approach point (MAPt) throughout the approach, and the lack of any form of vertical advisory guidance.
Additional CFIT investigations in Australia
The ATSB has investigated a number of other CFIT accidents in Australia, all involving aircraft that were not fitted with a TAWS. These included the following occurrences.
ATSB occurrence 200105769
On 10 December 2001, a Raytheon Beech 200C Super King Air was being operated under the IFR to Mt Gambier, South Australia, on an aeromedical flight with the pilot and a medical crew member on board. At approximately 2333 local time, the pilot reported to air traffic control that they were in the circuit at Mt Gambier and would report after landing. At approximately 2336, the aircraft impacted the ground at a position 3.1 NM from the threshold of the runway. The pilot was fatally injured and the medical crew member sustained serious injuries.
Dark night conditions existed in the area. The available evidence indicated that the pilot was conducting a GPS arrival procedure.
ATSB occurrence 200302172
On 15 May 2003, a Raytheon Beech 200C Super King Air was being operated under the IFR to Coffs Harbour, New South Wales, on an aeromedical flight with a pilot, 2 medical crew and a patient on board. The pilot conducted a missed approach from a low height and the aircraft impacted the sea, or a reef, approximately 3.2 NM north of the airport at about 0833 local time. During the missed approach, the aircraft narrowly avoided a breakwater and an adjacent restaurant. During the subsequent landing the aircraft was substantially damaged.
The pilot was conducting a GPS non-precision approach. Instrument meteorological conditions (IMC) existed at the time, including heavy rain and restricted visibility.
ATSB occurrence 200402797
On 28 July 2004, a Piper PA-31T Cheyenne, with one pilot and 5 passengers, was being operated on a private IFR flight from Bankstown, New South Wales, to Benalla, Victoria. The aircraft collided with terrain 18 NM south-east of Benalla. All occupants were fatally injured and the aircraft was destroyed.
IMC existed at the time and the pilot had reported commencing a GPS non-precision approach to Benalla.
ATSB occurrence 200503265
On 28 July 2004, a Piper PA31-350 Navajo Chieftain was being operated under the IFR to Mount Hotham, Victoria on a passenger charter flight with the pilot and 2 passengers on board. The aircraft collided with terrain 5 km south-east of the aerodrome and to the left of the extended centreline of runway. All occupants were fatally injured and the aircraft was destroyed.
IMC existed at the time and the pilot had reported commencing an RNAV GNSS approach.
Appendix G – Flight Safety Foundation CFIT Checklist
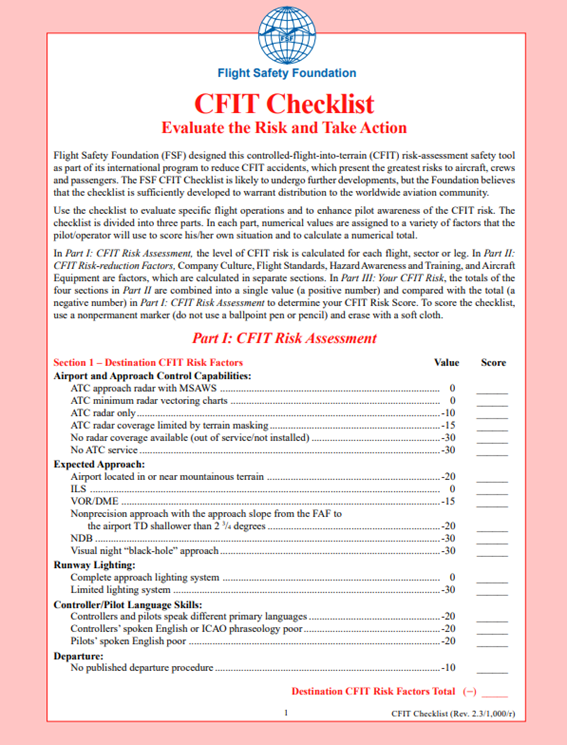
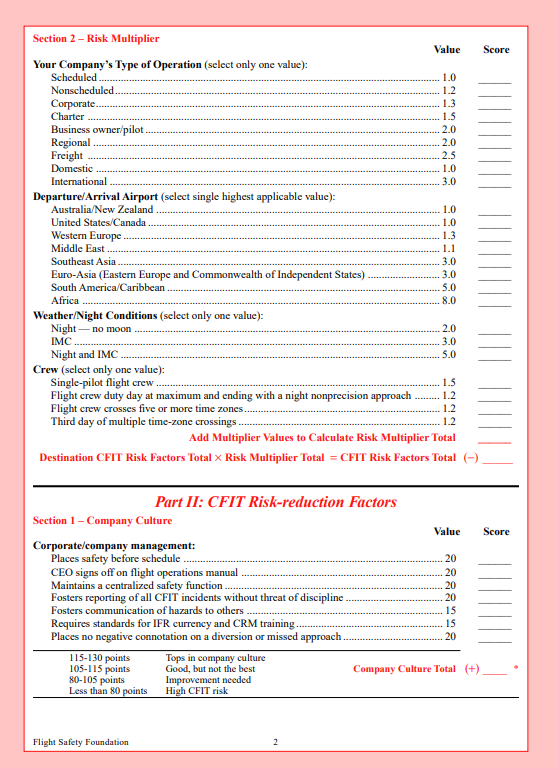
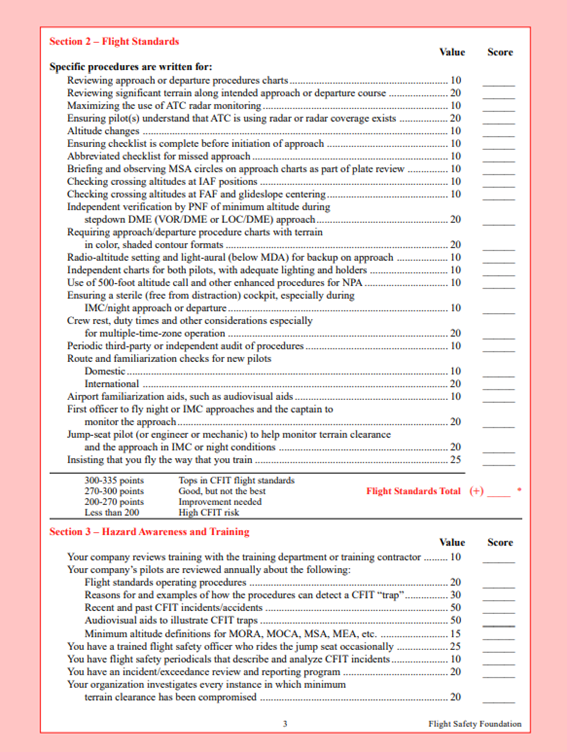
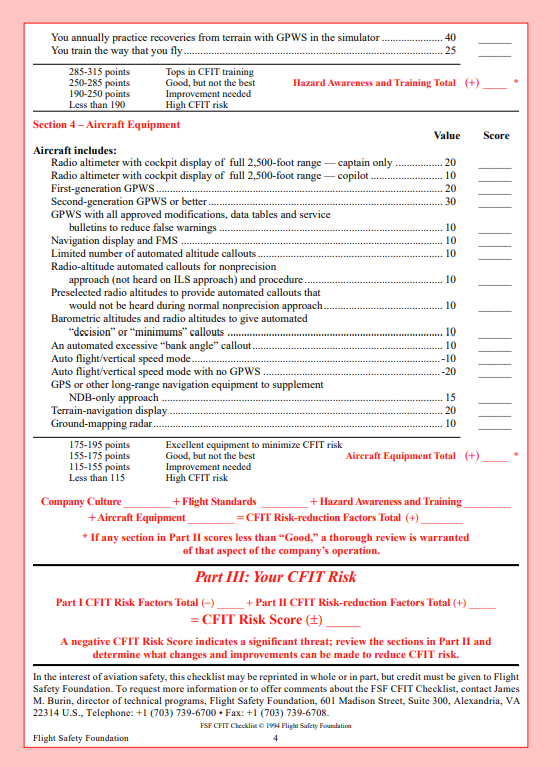
Purpose of safety investigationsThe objective of a safety investigation is to enhance transport safety. This is done through:
It is not a function of the ATSB to apportion blame or provide a means for determining liability. At the same time, an investigation report must include factual material of sufficient weight to support the analysis and findings. At all times the ATSB endeavours to balance the use of material that could imply adverse comment with the need to properly explain what happened, and why, in a fair and unbiased manner. The ATSB does not investigate for the purpose of taking administrative, regulatory or criminal action. TerminologyAn explanation of terminology used in ATSB investigation reports is available here. This includes terms such as occurrence, contributing factor, other factor that increased risk, and safety issue. Publishing informationReleased in accordance with section 25 of the Transport Safety Investigation Act 2003 Published by: Australian Transport Safety Bureau © Commonwealth of Australia 2022 Ownership of intellectual property rights in this publication Unless otherwise noted, copyright (and any other intellectual property rights, if any) in this report publication is owned by the Commonwealth of Australia. Creative Commons licence With the exception of the Coat of Arms, ATSB logo, and photos and graphics in which a third party holds copyright, this publication is licensed under a Creative Commons Attribution 3.0 Australia licence. Creative Commons Attribution 3.0 Australia Licence is a standard form licence agreement that allows you to copy, distribute, transmit and adapt this publication provided that you attribute the work. The ATSB’s preference is that you attribute this publication (and any material sourced from it) using the following wording: Source: Australian Transport Safety Bureau Copyright in material obtained from other agencies, private individuals or organisations, belongs to those agencies, individuals or organisations. Where you wish to use their material, you will need to contact them directly. |
[1] Instrument flight rules (IFR): a set of regulations that permit the pilot to operate an aircraft in instrument meteorological conditions (IMC), which have much lower weather minimums than visual flight rules (VFR). Procedures and training are significantly more complex as a pilot must demonstrate competency in IMC conditions while controlling the aircraft solely by reference to instruments. IFR-capable aircraft have greater equipment and maintenance requirements.
[2] Eastern Standard Time (EST): Coordinated Universal Time (UTC) + 10.0 hours.
[3] In the Lockhart River area, VHF contact with air traffic services was not generally available below 4,500 ft. Outside of VHF coverage, pilots communicated with Flightwatch using HF radio.
[4] Cloud cover: in aviation, cloud cover of the sky is reported using words/abbreviations that denote the extent of the cover. ‘Sky clear’ (SKC) indicates no cloud, ‘few’ (FEW) indicates 1–2 oktas (or eighths) is covered, ‘scattered’ (SCT) indicates 3–4 oktas is covered, ‘broken’ (BKN) indicates 5–7 oktas is covered, and ‘overcast’ (OVC) indicates that 8 oktas is covered.
[5] Landing minima: specified meteorological conditions of cloud ceiling and visibility. In order for an aircraft to land at an aerodrome, the actual weather conditions need to be at or above the landing minima.
[6] The ATSB obtained data broadcast by the automatic dependent surveillance broadcast (ADS-B) equipment fitted to the aircraft and GPS data transmitted from the pilot’s iPad with the OzRunways application installed. Further information on the aircraft’s recorded flight path during the 2 approaches is provided in Recorded flight data.
[7] 1 NM = 1.852 km
[8] The MDA published on the instrument approach chart was 830 ft and was based on the aerodrome forecast (TAF) QNH being set on the subscale of the aircraft’s pressure-sensitive altimeter. For this chart, the MDA could be reduced by 100 ft (to 730 ft) when using the actual QNH from an approved source. With the aircraft above the aerodrome and the actual QNH set, the altimeter indicates the approximate height above mean sea level. The aerodrome’s AWIS was an approved source for actual QNH and valid for a 15-minute period from the time of receipt.
[9] Flightwatch: on-request flight information service used to provide operational information including weather, aerodromes and navigational aids.
[10] VFR: a set of regulations that permit a pilot to operate an aircraft only in weather conditions generally clear enough to allow the pilot to see where the aircraft is going.
[11] The operator’s operations manual stated: ‘Due to the possibility of engine damage, asymmetric training in Company Cessna 404 aircraft is to be undertaken only where strictly necessary. Abrupt or large power changes are to be avoided where possible.’
[12] Instrument meteorological conditions (IMC): weather conditions that require pilots to fly primarily by reference to instruments, and therefore under instrument flight rules (IFR), rather than by outside visual reference. Typically, this means flying in cloud or limited visibility.
[13] The aerodrome forecast for that flight included periods of reduced visibility (3,000 m) in rain showers and cloud at 1,200 ft. Data from the aerodrome’s automatic weather station indicated the 1-minute visibility was 2,500 m at the airport when the aircraft passed the initial approach fix (IAF). However, the visibility had improved to 4,200 m by the time the aircraft reached the final approach fix (FAF) and continued to improve, to more than 10 km by the missed approach point (MAPt). There was no recorded rainfall during this period. The forecast and observed wind favoured a landing on runway 12.
[14] This aircraft retained the conventional airspeed indicator, altimeter and vertical speed indicator flight instruments, which were mounted in their usual positions relative to the ADI display. Those instruments were similar to the instruments fitted to VH-OZO.
[15] A number of other Cessna 404 aircraft introduced to Australia at about the same time also had modified seating arrangements with 11 passenger seats.
[16] An altitude alerting system provides an aural alert (tone) and/or a visual alert when an aircraft on climb/descent approaches the designated altitude and when deviating from that altitude during cruise. They are generally used to assist pilots monitor adherence to the ATC assigned level in controlled airspace, rather than mitigate the risk of controlled flight into terrain. Civil Aviation Order (CAO) 20.18 required aircraft conducting IFR operations in controlled airspace to have either an assigned altitude indicator or an altitude alerting system. For piston-engine aircraft, an altitude alerting system was only required for IFR operations above flight level 150 (which is 15,000 ft measured according to a standard atmosphere).
[17] Second CDI is partially hidden behind control column and clamp in Figure 8.
[18] The wide area augmentation system (WAAS) was developed by the US Federal Aviation Administration. The system provided augmentation information to GPS receivers, which improved the position accuracy and enabled localiser performance with vertical guidance (LPV) approaches to the runway. Those approaches took advantage of the increased position accuracy and were flown to a decision altitude, similar to a Category I instrument landing system procedure. The WAAS system covered most of the US, parts of Canada and Mexico.
[19] The default settings for the user-selectable fields were distance to the next waypoint, desired track, bearing to waypoint, groundspeed, ground track and estimated time en route.
[20] The navigation and terrain data were contained on 2 removable data cards, which slotted into the front face of each unit.
[21] One of the GNS 430W units was swapped with that from another aircraft in July 2018. The last terrain/obstacle database update of that unit was not determined.
[22] The series of Technical Standard Orders (TSO) C151 stipulated the minimum operational performance standards that a terrain awareness and warning system (TAWS) must meet to comply with regulatory requirements for the fitment and use of those systems.
[23] FLTA alerts were automatically inhibited when the aircraft was less than 200 ft above terrain while within 0.5 NM of the runway, or less than 125 ft above terrain within 1.0 NM of the runway.
[24] With approach flap selected, the inboard flap surface extended 10° and the outboard flap surface extended 8°.
[25] Vref: reference landing speed. It is normally defined as the speed required when crossing the runway threshold at 50 ft given the landing weight and configuration of the aircraft. It is usually calculated as 1.3 times the stalling speed in the landing configuration and at the prevailing aircraft weight.
[26] The operations manual used the term VAPP and Vref interchangeably. Often VAPP is used to refer to Vref plus additions for wind and other factors.
[27] Turbo-prop aeroplanes such as the SAAB 340 (maximum 37 passengers) and the Embraer EMB 120 (maximum 30 passengers) had a MTOW less than 15,000 kg.
[28] ICAO annexes specified standards and recommended practices (SARPS).
[29] In its notice of proposed amendment, the European Aviation Safety Agency (EASA) noted that the absence of a TAWS had been noted as a factor in 2 accidents in Europe for these types of aircraft in the previous 10 years. It also noted that new aeroplanes were already fitted with a TAWS and that a significant number of older aeroplanes had already been retrofitted with a TAWS or equivalent system. EASA also noted that the cost of fitting a TAWS in Europe was between €20,000 to €50,000.
[30] Transport Canada also noted that it had considered only introducing this requirement for turbine-engine aeroplanes (as per the US FAA) but that some stakeholders noted that some operators may discontinue using turbine-engine aeroplanes in favour of (less reliable) piston-engine aeroplanes to eliminate the cost of installing TAWS.
[31] MOPSC was defined at that time as the maximum passenger seat capacity of the aircraft, excluding pilot seats, flight deck seats and cabin crew seats.
[32] When the aeroplane was operated by a single pilot, this included the 11 passenger seats in the cabin and the front right seat, as that could also be used by a passenger. CASA further advised that an aeroplane with 2 seats at the front and 9 seats in the cabin (such as a Cessna 404 with the seating configuration specified in its type certificate data sheet) would be considered to have a MOPSC of 9 and the front right seat would not have to be removed.
[33] CASA advised that the definition of MOPSC referred to the number of passenger seats fitted to an aircraft available to be used by a passenger. An operator could not elect to limit the number of seats of an aircraft with a MOPSC of 10 or more through administrative or operational controls in order to have a MOPSC of 9 or less. Rather, seats would have to be physically removed from the aircraft.
[34] A SIGMET provides a concise description concerning the occurrence or expected occurrence, in areas over which meteorological watch is being maintained, of en-route weather phenomena that are potentially hazardous to aircraft.
[35] Visual meteorological conditions (VMC): an aviation flight category in which visual flight rules (VFR) flight is permitted – that is, conditions in which pilots have sufficient visibility to fly the aircraft while maintaining visual separation from terrain and other aircraft.
[36] In aviation meteorological products, time is expressed as coordinated universal time (UTC) and the data is generally expressed in coded terms. For ease of reference, the time has been converted to EST and the data has been decoded.
[37] In aerodrome forecasts and reports, the height datum for cloud is aerodrome elevation.
[38] TEMPO: a significant temporary variation from the prevailing conditions previously given in the TAF, expected to last for periods of between 30 and 60 minutes.
[39] Visibility reported in METARs/SPECIs was a 10-minute average.
[40] This improved the ceilometer’s response time to report changing conditions.
[41] In May 2012, BoM published a pamphlet Ceilometers and Visibility Meters as part of their aviation reference material series (available from www.bom.gov.au). The pamphlet provided information about the measurements made by these instruments and the associated advantages and limitations of the equipment.
[42] The term okta is used to refer to one eighth of cloud cover.
[43] From 9 September 2021, the naming of RNAV (GNSS) charts in Australia was progressively changed to RNP (required navigation performance) in alignment with an international convention.
[44] APV Baro-VNAV procedures required a navigation system capable of continuously computing a barometric VNAV path and displaying the relevant information on the instrument display.
[45] Civil Aviation Regulation 178 (Minimum height for flight under I.F.R.) stated that a pilot could not fly below a published lowest safe altitude except under certain conditions, such as during arrival if the aircraft was being flown in accordance with any instructions in the AIP, during an authorised instrument approach procedure, or if the aircraft was being flown by day in VMC.
[46] Availability of RAIM during the conduct of an RNAV GNSS approach provides an assurance of the integrity of the navigation system and that the calculated position is within the required tolerance for the procedure being flown.
[47] This paragraph and other AIP references were correct for the edition current at the time of the accident. That paragraph numbering may have changed during subsequent revisions of the publication.
[48] As discussed in the section GNS 430W overview, these receivers used satellite or ground-based augmentation (in regions where augmentation was available) to improve the position accuracy, enabling approaches providing localiser performance with vertical guidance (LPV) that could be flown to a decision altitude, similar to a Category I instrument landing system procedure.
[49] GPS units in the manufacturer’s 400 series without the ‘W’ designation (for example GPS 400, GNS 430/430A) used 0.3 NM CDI full-scale during the final approach segment. Those units could not be used to conduct LPV procedures.
[50] The approach procedure instructions published in the AIP to be established on the specified track before commencing descent were different to the navigation tolerances specified for the approach.
[51] For an aircraft conducting the Lockhart River RNAV (GNSS) runway 30 approach, descending on the recommended constant angle 3° profile, the aircraft crosses the FAF about 360 ft above the intermediate segment MSA.
[52] The CASA CNS/ATM resource kit (Chapter 9: Instrument flight rules operations, Flying the approaches), on the CASA website, also stated that ‘The tracking tolerance is half of full-scale deflection regardless of the CDI scale’. This resource kit content was based on the pre-December 2021 legislation but remained on the CASA website after December 2021.
[53] VAT is the indicated airspeed at the runway threshold (50 ft), which is equal to the stalling speed with landing gear extended and flaps in the landing position multiplied by 1.3. It is calculated at the aircraft’s maximum landing weight. At the maximum landing weight, VAT and Vref are the same.
[54] The aircraft was fitted with on-board Automatic Dependent Surveillance Broadcast (ADS-B) equipment, transmitting real-time operational data that enabled air traffic service providers to track aircraft. Airservices Australia recorded the transmissions received by its network of ADS-B receivers. That data could also be received by privately-operated equipment used to feed information to flight tracking websites.
[55] OzRunways is an electronic flight bag application that helps flight crew perform flight management tasks without the need for paper-based information. The OzRunways application also recorded flight data via a built-in GPS receiver. The data used in this investigation was transmitted from an iPad during the flight.
[56] 1 hPa difference equates to an altitude difference of about 30 ft.
[57] As previously noted, due to recorded altitude being truncated, this data have meant an altitude of 400–499 ft. The elevation of the terrain in the area was about 100 ft.
[58] According to the POH, the maximum gear extension speed was 182 kt, the maximum speed to select take-off and approach flap was 182 kt, and the maximum speed to select landing flap was 152 kt.
[59] Search and rescue time (SARTIME): the time nominated by a pilot for the initiation of search and rescue (SAR) action. If the pilot does not contact the SARTIME holder by the allotted time the search and rescue response will begin.
[60] The chief pilot advised the ATSB that, at some point prior to 29 October 2019, a Notice to Aircrew (number 13) was issued which outlined the operator’s change of requirements for OPCs to a period not exceeding 24 months.
[61] In this case, the pilot had conducted multiple flights under the IFR of over 1-hour duration in the last 6 months. On such flights, they had recorded a maximum of 30 minutes IFR flight time on these flights in their logbook.
[62] NOTAM: a notice distributed by means of telecommunication containing information concerning the establishment, condition or change in any aeronautical facility, service procedure or hazard, the timely knowledge of which is essential to personnel concerned with flight operations.
[63] The flight data used in this investigation was transmitted from the iPad during the approach.
[64] A descent rate of 600 ft/min requires a groundspeed of 113 kt to achieve a 3° approach path angle.
[65] CASA’s Air Operator’s Certificate Handbook: Volume 2 – Flying Operations stated in version 3.0 (November 2018) that ‘The use of the stabilised approach concept is mandatory for all approach operations.’ No further guidance regarding the contents of this guidance was included.
[66] The AIP also stated that, after passing the FAF, the descent rate ‘should not normally’ exceed 1,000 ft/min.
[67] ATSB Aviation Occurrence Report 200501977, Collision with Terrain, 11 km NW Lockhart River Aerodrome, 7 May 2005, VH-TFU, SA227-DC.
[68] ATSB Aviation Occurrence Investigation AO-2012-070, Unstable approach involving de Havilland Canada Dash 8, VH-XFZ, Laverton Aerodrome, WA, 17 May 2012.
[69] The ATSB also reviewed the recorded data for 2 other straight-in approaches that the pilot logged as RNAV GNSS approaches in September to October 2019. The recorded groundspeeds were 145–150 kt at the FAF, 140–155 kt at 1,000 ft and 115–135 kt at 300 ft. A full review of winds and indicated airspeeds was not conducted.
[70] ATSB Occurrence Report 200501977, Collision with Terrain, 11 km NW Lockhart River Aerodrome, 7 May 2005, VH-TFU, SA227-DC (Metro 23).
[71] In addition to these accidents, notable CFIT accidents during non-precision instrument approaches resulting in multiple fatalities also occurred on 11 June 1993 (Piper PA-31 piston-engine aircraft on scheduled passenger transport flight to Young, New South Wales, on an NDB/circling approach, 7 fatalities) and 27 April 1995 (Israel Aircraft Industries Westwind 1124 turbojet aircraft on scheduled freight flight to Alice Springs, Northern Territory, on a twin locator NDB approach, 3 fatalities).
[72] Between October 1999 and December 2000, there was a requirement for passenger transport operators under the IFR in turbine-engine aeroplanes with a MTOW more than 15,000 kg or carrying more than 9 passengers to conduct CFIT hazard awareness if the aircraft was not fitted with a GPWS or TAWS. After that time, all such aircraft were required to have a GPWS or TAWS. Some operators continued to include CFIT hazard awareness training as a requirement in their operations manual. Further details are provided in ATSB Occurrence Report 200501977.
[73] The operator’s operations manual also stated: ‘Extreme caution should be exercised in low visibility operations. Note that when encountering low visibility conditions due to rain, it is important to be aware that an illusion of being too high can occur, with a resulting undershoot being a possibility.’
[74] The MOPSC for an Australian operator was defined as the maximum passenger seat capacity of the aircraft approved by CASA as part of the approval of the operator’s exposition under CASR Part 119 and specified in the operator’s operations manual.
[75] That is, aeroplanes certified to be operated by a single pilot in accordance with the type certificate data sheet and whose flight manual provided that the flight crew could consist of a single pilot.
[76] A drum pointer altimeter has a single pointer that indicates tens and hundreds of feet, while a single drum indicates thousands and tens-of-thousands of feet (and fractions thereof) in numerals. A counter-drum pointer altimeter includes a single pointer that indicates tends and hundreds of feet while one or more coupled drums (or counters) indicated hundreds, thousands and tens-of-thousands of feet.
[77] Previous research conducted by Fitts and Jones (1947) also identified similar problems with misreading of 3-pointer altimeters, particularly in terms of misreading by 1,000 ft and misreading by 10,000 ft. They found that misreading of altimeters was the most common error in interpreting aircraft instruments at that time.
[78] The same exclusion for 3-pointer and drum-pointer altimeters was not stated in ICAO Annex 6 Part II International General Aviation – Aeroplanes.
[80] 1 hPa difference equates to an altitude difference of about 30 ft.